Advertisement
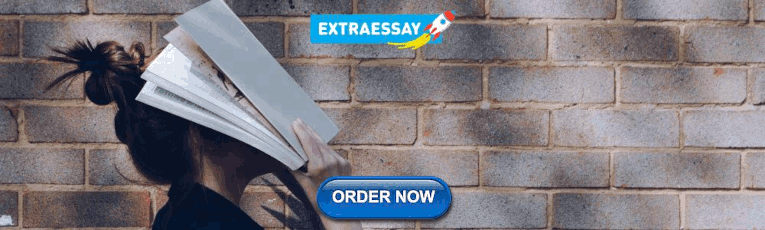
Jatropha curcas : a review on biotechnological status and challenges
- Review Article
- Published: 22 April 2011
- Volume 5 , pages 197–215, ( 2011 )
Cite this article
- Priyanka Mukherjee 1 ,
- Alok Varshney 2 ,
- T. Sudhakar Johnson 2 &
- Timir Baran Jha 1
1392 Accesses
3 Altmetric
Explore all metrics
Plant tissue culture and molecular biology techniques are powerful tools of biotechnology that can complement conventional breeding, expedite crop improvement and meet the demand for availability of uniform clones in large numbers. Jatropha curcas Linn., a non-edible, eco-friendly, non-toxic, biodegradable fuel-producing plant has attracted worldwide attention as an alternate sustainable energy source for the future. This review presents a consolidated account of biotechnological interventions made in J. curcas over the decades and focuses on contemporary information and trends of future research.
This is a preview of subscription content, log in via an institution to check access.
Access this article
Subscribe and save.
- Get 10 units per month
- Download Article/Chapter or eBook
- 1 Unit = 1 Article or 1 Chapter
- Cancel anytime
Price includes VAT (Russian Federation)
Instant access to the full article PDF.
Rent this article via DeepDyve
Institutional subscriptions
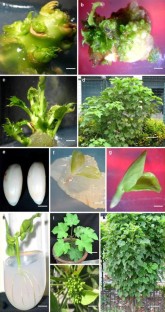
Similar content being viewed by others
Plant Regeneration and Genetic Transformation in Jatropha
Genetic Transformation and Transgenics of Jatropha curcas, a Biofuel Plant
Somatic Embryogenesis in Jatropha curcas
Adholeya A, Singh R (2006) Quality considerations in Jatropha curcas. In: Bhojvaid PP (ed) Biofuels: towards a greener and secure energy future. TERI Press, New Delhi, pp 173–180
Azam MM, Waris A, Nahar NM (2005) Prospects and potential of fatty acid methyl esters of some non-traditional seed oils for use as biodiesel in India. Biomass Bioenergy 29:293–302
Google Scholar
Basha SD, Sujatha M (2007a) Genetic analysis of Jatropha species and interspecific hybrids of Jatropha curcas using nuclear and organelle specific markers. Euphytica 168:197–214
Basha SD, Sujatha M (2007b) Inter and intra-population variability of Jatropha curcas (L.) characterized by RAPD and ISSR markers and development of population-specific SCAR markers. Euphytica 156:375–386
CAS Google Scholar
Basha SD, Franis G, Makkar HPS, Becker K, Sujatha M (2009) A comparative study of biochemical traits and molecular markers for assessment of genetic relationships between Jatropha curcas L. germplasm from different countries. Plant Sci 176:812–823
Becker EW (1994) Microalgae: biotechnology and microbiology. Cambridge University Press, Cambridge
Bhansali R (1990) Somatic embryogenesis and regeneration of plantlets in pomegranate. Ann Bot 66:249–254
Boora KS, Dhollin RS (2010) Evaluation of genetic diversity in Jatropha curcas L. using RAPD markers. Ind J Biotech 9:50–57
Cabral GB, Aragao FJL, Matsumoto K, Monte Neshich DC, Rech EL (1992) First international scientific meeting of the Cassava. Biotechnology Network (CBN) Cartagena Colombia, Abstracts: 48
Caetano-Anolles G, Gresshoff PM (1997) DNA markers protocols applications and overviews. Wiley-Liss, New York
Cahoon EB (2003) Genetic enhancement of soybean oil for industrial uses: prospects and challenges. AgBioForum 6:11–13
Canter PH, Thomas H, Ernst E (2005) Bringing medicinal plants into cultivation: opportunities and challenges for biotechnologies. Trends Biotechnol 23:180–185
PubMed CAS Google Scholar
Carron MP, Enjalric F (1982) Studies on vegetative propagation of Hevea brasiliensis by somatic embryogenesis and in vitro Microcutting. In: Fujiwara A (ed) Plant tissue culture, Maruzen, Tokyo, pp 751–752
Carron MP, Deschamps A, Enjalric F, Lardet L (1985) Vegetative in vitro propagation by microcuttings of selected rubber tree: a wide spread technique before 2000. In: Proceedings of 1st international rubber tissue culture workshop, Kuala Lumpur, pp 22–26
Carron MP, Etienne H, Lardet L, Campagha S, Persin Y, Leconte A, Chaine C (1995) Somatic embryogenesis in Rubber ( Hevea brasiliensis Mull.-Arg.) In: Jain SM, Gupta P, Newton R (eds) Somatic embryogenesis in woody plants, vol 2. Kluwer, Dordrecht, pp 117–136
Carvalho CR, Clarindo WR, Praça MM, Araújo FS, Carels N (2008) Genome size base composition and karyotype of Jatropha curcas L., an important biofuel plant. Plant Sci 174:613–617
Chan K (2003) Some aspects of toxic contaminants in herbal medicines. Chemosphere 52:1361–1371
Cristi Y (2007) Biodiesel from microalgae. Biotech Adv 25:294–306
D’Amato F (1975) The problem of genetic stability in plant tissue and cell cultures. In: Frankel OH, Hawkes JG (eds) Crop genetic resources for today and tomorrow. Cambridge University Press, Cambridge, pp 338–348
Da Camara Machado A, Frick NS, Kremen R, Katinger H, da Camara Machado ML (1997) Biotechnological approaches to the improvement of Jatropha curcas . In Proceedings of the International Symposium on Jatropha , Nicaragua
Dahmer N, Wittmann MTS, dos Santos Dias LA (2009) Chromosome numbers of Jatropha curcas L.: an important agrofuel plant. Crop Breed Appl Biotechnol 9:386–389
Dandekar AM (2003) Techniques for manipulating quality and productivity traits in horticultural crops. Acta Hort 625:293–305
Das P, Rout GR, Das AB (1993) Somatic embryogenesis in callus cultures of Mussaenda erythrophylla cvs. Plant Cell Tissue Organ Cult 35:199–201
Datta MM, Mukherjee P, Ghosh B, Jha TB (2007) In vitro clonal propagation of biodiesel plant ( Jatropha curcas L.) Curr Sci 93:1438–1442
De Jong AJ, Schmidt EDL, de Vries SC (1993) Early events in higher plant embryogenesis. Plant Mol Biol 22:367–377
PubMed Google Scholar
Dehgan B (1984) Phylogenetic significance of interspecific hybridization in Jatropha (Euphorbiaceae). Syst Bot 9:467–478
Dehgan B, Webster GL (1979) Morphology and infrageneric relationships of the Genus Jatropha (Euphorbiaceae). Univ California Pub Bot 74:67–73
Demirbas A (2003) Chemical and fuel properties of seventeen vegetable oils. Energy Source 25:721–728
Demirbas A (2006) Energy priorities and new energy strategies. Energy Edu Sci Technol 16:53–109
Deore A, Johnson TS (2008a) Occurrence of vivipary in Jatropha curcas L. Curr Sci 95:321–322
Deore A, Johnson TS (2008b) High-frequency plant regeneration from leaf-disc cultures of Jatropha curcas L.: an important biodiesel. Plant Biotechnol Rep 2:7–11
Divakara BN, Upadhyaya HD, Wani SP, Laxmipathi Gowda CL (2010) Biology and genetic improvement of Jatropha curcas L.: a review. Appl Energy 87:732–742
Dudits D, Bogre L, Gyorgyey J (1991) Molecular and cellular approaches to the analysis of plant embryo development from somatic cells in vitro. J Cell Sci 99:475–484
Edem DO (2002) Palm oil: biochemical, physiological, nutritional, hematological, and toxicological aspects: a review. Plant Foods Hum Nutr 57:319–341
Fedorov A (1969) Chromosome numbers of flowering plants. Academy of Sciences of the USSR, Leningrad
Filho G, Rocha N, Brodzki D, Djega-Mariadassou G (1992) Formation of alkanes, alkylcycloalkanes and alkylbenzenes during the catalytic hydrocracking of vegetable oils. Fuel 72(4):543–549
Francis G, Edinger R, Becker K (2005) A concept for simultaneous wasteland reclamation, fuel production, and socioeconomic development in degraded areas in India: need, potential and perspectives of Jatropha plantations. Nat Resour Forum 29:12–24
Gamborg OL, Miller RA, Ojima K (1968) Nutrient requirements of suspension cultures of soybean root cells. Exp Cell Res 50(1):151–158
Ganesh RS, Parthiban KT, Senthil KR, Thiruvengadam V, Paramathma M (2008) Genetic diversity among Jatropha species as reveled by RAPD markers. Genet Resour Crop Evol 55:803–809
George EF, Hall M A, De Klerk GJ (2008) Plant propagation by tissue culture. The background, 3rd edn, vol 1. Springer, Berlin
Gunstone FD (2004) Rapeseed and Canola Oil: Production, Processing, properties and uses. Blackwell, London
Gupta S, Srivastava M, Mishra GP, Naik PK, Chauhan RS, Tiwari SK, Kumar M, Singh R (2008) Analogy of ISSR and RAPD markers for comparative analysis of genetic diversity among different Jatropha curcas genotypes. Afr J Biotech 7(23):4230–4243
Haberlandt G (1902) Culturversuche mit isolierten. Pflanzenzellen Sitzungsber Akad Wiss Wien Math Nat Cl III Abt 1:69-91
IPCN (2009) Index to Plant Chromosome Numbers. Available at: http://mobot.mobot.org/W3T/Search/ipcn.html
Isigigur A, Karaosmonoglu F, Aksoy HA (1994) Methyl ester from safflower seed oil of Turkish origin as a biofuel for diesel engines. Appl Biochem Biotechnol 45:103–112
Jha TB, Mukherjee P, Datta MM (2007) Somatic embryogenesis in Jatropha curcas Linn., an important biofuel plant. Plant Biotech Rep 1:135–140
Jin S, Zhang X, Liang S, Nie Y, Guo, Huang C (2005) Factors affecting transformation efficiency of embryogenic callus of upland cotton ( Gossypium hirsutum ) with Agrobacterium tumefaciens . Plant Cell Tissue Organ Cult 81:229–237
Jones N, Miller JH (1992) Jatropha curcas —a multipurpose species for problematic sites. Land Resour Ser 1:1–12
Jongschaap REE, Corré WJ, Bindraban PS, Brandenburg WA (2007) Claims and facts on Jatropha curcas L. Wageningen, The Netherlands: Plant Research International. http://www.factfuels.org/media_en/Claims_and_Facts_on_Jatropha_WUR?session=isgsklbna58j7grrfst888n5r7
Joshi K, Chavan P, Warude D, Patwardhan P (2004) Molecular markers in herbal drug technology. Curr Sci 87:159–165
Kaewpoo M, Te-Chato S (2010) Study on ploidy level of micropropagated Jatropha curcas L. via flow cytometry. J Agric Tech 6:391–400
Kalimuthu K, Paulsamy S, Senthilkumar R, Sathya M (2007) In vitro propagation of the biodiesel plant Jatropha curcas L. Plant Tissue Cult Biotech 17:137–147
Kalscheuer R, Luftmann H, Steinbüchel A (2004) Synthesis of novel lipids in Saccharomyces cerevisiae by heterologous expression of an unspecific bacterial acyltransferase. Appl Environ Microbiol 70:7119–7125
Kalscheuer R, Stöl T, Steinbüchel A (2006) Microdiesel: Escherichia coli engineered for fuel production. Microbiology 152:2529–2536
Karmee SK, Chadha A (2005) Preparation of biodiesel from crude oil of Pongamia pinnata . Bioresour Technol 96:1425–1429
Khemkladngoen N, Caragena J, Shibagaki N, Fukui K (2011) Adventitious shoot regeneration from juvenile cotyledons of a biodiesel producing plant, Jatropha curcas L. J Biosci Bioeng 111:67–70
Khurana-Kaul V, Kachhwaha S, Kothari SL (2010) Direct shoot regeneration from leaf explants of Jatropha curcas in response to thidiazuron and high copper contents in the medium. Biol Plant 54:369–372
Komamine A, Murata N, Nomura K (2005) Mechanisms of somatic embryogenesis in carrot suspension cultures—morphology, physiology, biochemistry, and molecular biology. In Vitro Cell Dev Biol Plant 41:6–10
Kothari SL, Varshney A (1998) Morphogenesis in long term maintained immature embryo derived callus of wheat ( Triticum aestivum L.)—histological evidence for both somatic embryogenesis and organogenesis. Plant Biochem Biotech 7:93–98
Kumar J, Gupta PK (2008) Molecular approaches for improvement of medicinal and aromatic plants. Plant Biotech Rep 2:93–112
Kumar N, Reddy MP (2010) Plant regeneration through the direct induction of shoot buds from petiole explants of Jatropha curcas : a biofuel plant. Ann Appl Biol 156:367–375
Kumar A, Sharma S (2008) An evaluation of multipurpose oil seed crop for industrial uses ( Jatropha curcas L.). A review. Ind Crop Prod 28:1–10
Kumar RV, Tripathi YK, Shukla P, Ahlawat SP, Gupta VK (2009) Genetic diversity and relationships among germplasm of Jatropha curcas L, revealed by RAPDs. Trees 23:1075–1079
Kumar N, Vijay Anand KG, Reddy MP (2010a) Shoot regeneration from cotyledonary leaf explants of Jatropha curcas : a biodiesel plant. Acta Physiol Plant 32:917–924
Kumar N, Vijay Anand KG, Sudheer Pamidimarri DVN, Sarkar T, Reddy MP, Radhakrishnan T, Kaul T, Reddy MK, Sopori SK (2010b) Stable genetic transformation of Jatropha curcas via Agrobacterium tumefaciens -mediated gene transfer using leaf explants. Ind Crop Prod 32:41–47
Kumar N, Vijay Anand KG, Reddy MP (2011) In vitro regeneration from petiole explants of non-toxic Jatropha curcas . Ind Crop Prod 33:146–151
Levine M (1947) Differentiation of carrot root tissue grown in culture. Bull Torrey Bot Club 74:321
Li MR, Li HQ, Wu GJ (2006a) Study on factors influencing Agrobacterium -mediated transformation of Jatropha curcas . Fen Zi Xi Bao Sheng Wu Xue Bao 39:83–89
Li ZT, Dhekeny S, Dutt M, Van Anan M, Tattersll MJ, Kelley KT, Gray J (2006b) Optimizing Agrobacterium -mediated transformation of grapevine. In Vitro Cell Dev Biol Plant 42:220–227
Li M, Li H, Jiang H, Pan X, Wu G (2008) Establishment of an Agrobacterium mediated cotyledon disc transformation method for J. curcas . Plant Cell Tissue Organ Cult 92:173–181
Litz RE, Gray DJ (1995) Somatic embryogenesis for agricultural improvement. World J Microbiol Biotech 11:416–425
Liu W, Xu Z, Chua N (1993) Auxin polar transport is essential for the establishment of bilateral symmetry during early plant embryogenesis. Plant Cell 5:621–630
Lo Schiavo F (1989) DNA methylation of embryogenic carrot cell cultures and its variations as caused by mutation, differentiation, hormones and hypomethylating drugs. Theor Appl Genet 77:325–331
Lohia AK (2006) Jatropha for sustainable employment, energy security, and for checking soil erosion. In: Bhojvaid PP (ed) Biofuels: towards a greener and secure energy future. TERI Press, New Delhi, pp 255–262
Ma F, Hanna MA (1999) Biodiesel production: a review. Bioresour Technol 70:1–15
Mandal R, Mithra P (2006) The Indian biofuels programme: the National Mission on Bio-diesel. In: Bhojvaid PP (ed) Biofuels: towards a greener and secure energy future. TERI Press, New Delhi, pp 13–22
Martin KP (2003) Plant regeneration through direct somatic embryogenesis on seed coat explants of cashew ( Anacardium occidentale L.). Sci Hort 98:299–304
Miao X, Wu Q (2004) High yield bio-oil production from fast pyrolysis by metabolic controlling of Chlorella protothecoides . J Biotech 110:85–93
Michaux-Ferriere N, Grout H, Carron MP (1992) Origin and ontogenesis of somatic embryos in Hevea brasiliensis (Euphorbiaceae). Am J Bot 79:174–180
Mittelbach M, Remschmidt C (2004) Biodiesel: the comprehensive handbook. Boersedruck, Vienna
Montes LR, Azurdia C, Jongschaap REE, Van Loo EN, Barillas E, Visser R, Mejia L (2008) Global evaluation of genetic variability in Jatropha curcas . http://www.pri.wur.nl/NR/rdonlyres/90AF26A1-47D5-4F2F-9E96-D413C2933685/70112/PosterMontesHR.pdf
Mukherjee P, Jha TB (2009) Biotechnological improvement of a biofuel crop— Jatropha curcas . In: Wright JH, Evans DA (eds) New research in biofuels. NOVA Science Publishers, New York, pp 31–52
Murashige T, Skoog F (1962) A revised medium for rapid growth and bioassays with tobacco tissue culture. Physiol Plant 15:473–479
Pamidiamarri DVNS, Pandya N, Reddy MP, Radhakrishnan T (2008) Comparative study of interspecific genetic divergence and phylogenic analysis of genus Jatropha by RAPD and AFLP: genetic divergence and phylogenic analysis of genus Jatropha . Mol Biol Rep 36(5):901–907
Pax F (1910) Euphorbiaceae—Jatropheae. In: Engler A (ed) Das Pflanzenreich IV. Verlag on Wilhelm Engelmann, Leipzig
Pierik RLM (1991) Commercial aspects of micropropagation. In: Prakash J, Pierik RLM (eds) Horticulture—new technologies and applications. Kluwer, Dordrecht, pp 141–153
Pinto AC, Guarieiro LLN, Rezende MJC, Ribeiro NM, Torres EA, Lopes WA, Pereira PAP, Andrade JB (2005) Biodiesel: An overview. J Brazil Chem Soc 16:1313–1330
Prabakaran AJ, Sujatha M (1999) Jatropha tanjorensis Ellis & Saroja, a natural interspecific hybrid occurring in Tamil Nadu, India. Genet Resour Crop Evol 46:213–218
Purkayastha J, Sugla T, Paul A, Solleti SK, Mazumdar P, Basu A, Mohommad A, Ahmed Z, Sahoo L (2010) Efficient in vitro plant regeneration from shoot apices and gene transfer by particle bombardment in Jatropha curcas . Biol Planta 54(1):13–20
Qi-Bao Sun, Lin-Feng Li, Yong Li, Guo-Jiang Wu, Xue-Jun Ge (2008) SSR and AFLP markers reveal low genetic diversity in the biofuel plant Jatropha curcas in China. Crop Sci 48:1865–1871
Qin J (2005) Bio-hydrocarbons from algae, Report for the Rural Industries Research and Development Corporation, RIRDC Publication No. 05/025
Qin WL, Yi Wei-DaL, Shu-Lin P, Ying XU, Lin T, Fang C (2004) Plant regeneration from epicotyl explants of Jatropha curcas . J Plant Physiol Mol Biol 30:475–478
Raemakers CJJM, Bessembinder JJE, Staritsky G, Jacobsen E, Visser RGF (1993a) Induction, germination and shoot development of somatic embryos in Cassava. Plant Cell Tissue Organ Cult 33:151–156
Raemakers CJJM, Schavemaker CM, Jacobsen E, Visser RGE (1993b) Improvements of cyclic somatic embryogenesis of Cassava ( Manihot esculenta Crantz). Plant Cell Rep 12:226–229
Raemakers KJJM, Jacobsen E, Visser RGF (1999) Direct cyclic somatic embryogenesis of Cassava for production purposes. Plant Cell Cult Protocols 111:61–70
Raemakers K, Jacobsen E, Visser R (2000) The use of somatic embryogenesis for plant propagation in Cassava. Mol Biotech 14:215–221
Rajore S, Batra A (2005) Efficient plant regeneration via shoot tip explant in Jatropha curcas . J Plant Biochem Biotech 14:73–75
Ramadhas AS, Jayaraj S, Muraleedharan C (2004a) Biodiesel production from high FFA rubber seed oil. Fuel 84:335–340
Ramadhas AS, Jayaraj S, Muraleedharan C (2004b) Use of vegetable oils as I.C. engine fuels—a review. Renew Energy 29:727–742
Ranade SA, Srivastava AP, Rana TS, Srivastava J, Tuli R (2008) Easy assessment of diversity in Jatropha curcas L. plants using two single-primer amplification reaction (SPAR) methods. Biomass Bioenergy 32:533–540
Reddy MP, Chikara J, Patolia JS, Ghosh A (2007) Genetic improvement of Jatropha curcas adaptability and oil yield In: FACT SEMINAR on Jatropha curcas L. agronomy and genetics. FACT Foundation, Wageningen
Reddy MP, Kumar N, Vijay Anand KG, Singh AH, Singh S (2008) Method for micropropagation of Jatropha curcas plants from leaf explants (Patent filed US and PCT, File No. 2537de2008)
Reinert J (1958) Morphogenese und ihre Kotrolle an Gewebekulturen aus Corotten. Naturwiss 45:344
Richards CL, Bossdorf O, Muth NZ, Gurevitch J, Pigliucci M (2006) Jack of all trades, master of some; on the role of phenotypic plasticity in plant invasions. Eco Lett 9:981–993
Sardana J, Batra A, Ali DJ (2000) An expeditious method for regeneration of somatic embryos in Jatropha curcas L. Phytomorphology 50:239–242
Schmidt EDL, Guzzo F, Toonen MAJ, de Vries SC (1997) A leucine-rich repeat containing receptor-like kinase marks somatic plant cells competent to form embryos. Development 124:2049–2062
Senthil Kumar R, Parthiban KT, Govinda Rao M (2009) Molecular characterization of Jatropha genetic resources through inter-simple sequence repeat (ISSR) markers. Mol Biol Rep 36:1951–1956
Shay EG (1993) Diesel fuel from vegetable oils: status and opportunities. Biomass Bioenergy 4:227–242
Shrivastava S, Banerjee M (2008) In vitro clonal propagation of physic nut ( Jatropha curcas L): Influence of additives. Int J Integrative Biol 3:73–79
Singh A, Reddy MP, Chikara J, Singh S (2010) A simple regeneration protocol from stem explants of Jatropha curcas —a biodiesel plant. Ind Crops Prod 31:209–213
Soomro R, Memon RA (2007) Establishment of callus and suspension culture in Jatropha curcas . Pak J Bot 39:2431–2441
Soontornchainaksaeng P, Jenjittikul T (2003) Karyology of Jatropha (Euphorbiaceae) in Thailand. Thai For Bull 31:105–112
Stamp JA (1987) Somatic embryogenesis in Cassava: the anatomy and morphology of the regeneration process. Ann Bot 59:451–459
Stamp JA, Henshaw GG (1982) Somatic embryogenesis in Cassava. Zpflanzenphysiology 105:183–187
Stamp JA, Henshaw GG (1987) Somatic embryogenesis from clonal leaf tissue of Cassava. Ann Bot 59:445–450
Steward FC (1958) Growth and development of cultured cells. III. Interpretations of the growth from cell to carrot plant. Am J Bot 45:709–713
Strasburger E (1878) Uber Polyembryonie Jenaische Z. Naturwiss 12:64
Subramanyam K, Muralidhararao D, Devanna N (2009) Genetic diversity assessment of wild and cultivated varieties of Jatropha curcas (L.) in India by RAPD analysis. Afr J Biotech 8:1900–1910
Sujatha M, Mukta N (1996) Morphogenesis and plant regeneration from tissue cultures of Jatropha curcas . Plant Cell Tissue Organ Cult 44:135–141
Sujatha M, Sivaraj N, Prasad MS (2000) Biochemical and histological changes during in vitro organogenesis in Jatropha integerrima . Biol Plant 43:167–171
Sujatha M, Makkar HPS, Becker K (2005) Shoot bud proliferation from axillary nodes and leaf sections of non-toxic Jatropha curcas L. Plant Growth Reg 47:83–90
Sujatha M, Reddy TP, Mahasi MJ (2008) Role of biotechnological interventions in the improvement of castor ( Ricinus communis L.) and Jatropha curcas L. Biotech Adv 26:424–435
Sun Q-B, Li L-F, Li Y, Wu G-J, Ge X-J (2008) SSR and AFLP 959 markers reveal low genetic diversity in the biofuel plant Jatropha curcas in China. Crop Sci 48:1865–1871
Szabados L, Hoyos R, Roca W (1987) In vitro somatic embryogenesis and plant regeneration of Cassava. Plant Cell Rep 6:248–251
Tatikonda L, Wani SP, Kannan S, Beerelli N, Sreedevi TK, Hoisington DA, Devi P, Varshney RK (2009) AFLP based molecular characterization of an elite germplasm collection of Jatropha curcas , a biofuel plant. Plant Sci 176:505–513
Thepsamran N, Thepsithar C, Thongpukdee A (2007) In vitro multiple shoot induction of physic nut ( Jatropha curcas ). http://www.scisoc.or.th/stt/32/sec_f/paper/stt32_F_F0007.pdf
Tong L, Peng SM, Deng WY, Ma DW, Xu Y, Xiao M (2006) Characterization of a new stearoyl-acyl carrier protein desaturase gene from Jatropha curcas . Biotech Lett 28:657–662
Trivedi S, Gaudani H, Gupta M, Gupta N, Patil P, Krishna VK, Reddy MP (2009) Establishment of Agrobacterium -mediated genetic transformation in Jatropha curcas L. Int J Agric Sci 1(2):11–20
Varshney A, Johnson TS (2010) Efficient plant regeneration from immature embryo cultures of Jatropha curcas , a biodiesel plant. Plant Biotech Rep 4:139–148
Varshney A, Sangapillai R, Patil M, Johnson TS (2011) Histological evidence of morphogenesis from various explants of Jatropha curcas L. Trees. doi: 10.1007/s00468-011-0546-x
Warakagoda PS, Subasinghe S (2009) In vitro culture establishment and shoot proliferation of Jatropha curcas L. Trop Agric Res Ext 12:77–80
Witkowska M, Ohmido Nobuko, Cartagena J, Shibagaki N, Kajiyama S, Fukui K (2009) Physical mapping of ribosomal DNA genes on Jatropha curcas chromosomes by multicolour FISH. Cytologia 74(2):133–139
Yang X, Zhang X (2010) Regulation of somatic embryogenesis in higher plants. Crit Rev Plant Sci 29(1):36–57
Ying Z, Yunxiao W, Luding J, Ying X, Yingchun W, Daihua L (2007) Aquaporin JcPIP2 is involved in drought responses in Jatropha curcas . Acta Biochem Biophys Sin 39:787–794
Zhang F, Niu B, Wang Y, Chen F, Wang S, Xu Y (2008) A novel betaine aldehyde dehydrogenase gene from Jatropha curcas , encoding an enzyme implicated in adaption to environmental stress. Plant Sci 174:510–518
Download references
Author information
Authors and affiliations.
Plant Biotechnology Laboratory, Department of Botany, Presidency University, Kolkata, 700 073, India
Priyanka Mukherjee & Timir Baran Jha
Plant Metabolic Engineering Group, Reliance Life Sciences Pvt. Ltd., Dhirubhai Ambani Life Sciences Centre, Navi Mumbai, 400 701, India
Alok Varshney & T. Sudhakar Johnson
You can also search for this author in PubMed Google Scholar
Corresponding authors
Correspondence to T. Sudhakar Johnson or Timir Baran Jha .
Additional information
P. Mukherjee and A. Varshney contributed equally.
Rights and permissions
Reprints and permissions
About this article
Mukherjee, P., Varshney, A., Johnson, T.S. et al. Jatropha curcas : a review on biotechnological status and challenges. Plant Biotechnol Rep 5 , 197–215 (2011). https://doi.org/10.1007/s11816-011-0175-2
Download citation
Received : 18 March 2011
Accepted : 30 March 2011
Published : 22 April 2011
Issue Date : July 2011
DOI : https://doi.org/10.1007/s11816-011-0175-2
Share this article
Anyone you share the following link with will be able to read this content:
Sorry, a shareable link is not currently available for this article.
Provided by the Springer Nature SharedIt content-sharing initiative
- Crop improvement
- In vitro plant regeneration
- Jatropha curcas
- Molecular markers
- Organogenesis
- Somatic embryogenesis
- Find a journal
- Publish with us
- Track your research
An official website of the United States government
Official websites use .gov A .gov website belongs to an official government organization in the United States.
Secure .gov websites use HTTPS A lock ( Lock Locked padlock icon ) or https:// means you've safely connected to the .gov website. Share sensitive information only on official, secure websites.
- Publications
- Account settings
- Advanced Search
- Journal List

Bioactive Compounds and Biological Activities of Jatropha curcas L. Kernel Meal Extract
Ehsan oskoueian, norhani abdullah, syahida ahmad, wan zuhainis saad, abdul rahman omar.
- Author information
- Article notes
- Copyright and License information
Author to whom correspondence should be addressed; E-Mail: [email protected] ; Tel.: +60-389466700; Fax: +60-389430913.
Received 2011 May 24; Revised 2011 Jul 20; Accepted 2011 Aug 22; Collection date 2011.
This article is an open-access article distributed under the terms and conditions of the Creative Commons Attribution license ( http://creativecommons.org/licenses/by/3.0/ ).
Defatted Jatropha curcas L. ( J. curcas ) seed kernels contained a high percentage of crude protein (61.8%) and relatively little acid detergent fiber (4.8%) and neutral detergent fiber (9.7%). Spectrophotometric analysis of the methanolic extract showed the presence of phenolics, flavonoids and saponins with values of 3.9, 0.4 and 19.0 mg/g DM, respectively. High performance liquid chromatography (HPLC) analyses showed the presence of gallic acid and pyrogallol (phenolics), rutin and myricetin (flavonoids) and daidzein (isoflavonoid). The amount of phorbol esters in the methanolic extract estimated by HPLC was 3.0 ± 0.1 mg/g DM. Other metabolites detected by GC-MS include: 2-(hydroxymethyl)-2 nitro-1,3-propanediol, β-sitosterol, 2-furancarboxaldehyde, 5-(hydroxymethy) and acetic acid in the methanolic extract; 2-furancarboxaldehyde, 5-(hydroxymethy), acetic acid and furfural (2-furancarboxaldehyde) in the hot water extract. Methanolic and hot water extracts of kernel meal showed antimicrobial activity against both Gram positive and Gram negative pathogenic bacteria (inhibition range: 0–1.63 cm) at the concentrations of 1 and 1.5 mg/disc. Methanolic extract exhibited antioxidant activities that are higher than hot water extract and comparable to β-carotene. The extracts tended to scavenge the free radicals in the reduction of ferric ion (Fe 3+ ) to ferrous ion (Fe 2+ ). Cytotoxicity assay results indicated the potential of methanolic extract as a source of anticancer therapeutic agents toward breast cancer cells.
Keywords: Jatropha curcas L., bioactive compounds, antibacterial, antioxidant, cytotoxicity, kernel meal
1. Introduction
Jatropha curcas L. ( J. Curcas ) belongs to the Euphorbiaceae family and grows in tropical and sub-tropical regions like Central and South America, Africa, India and South East Asia [ 1 ]. It is a multipurpose plant with several industrial and medicinal applications. Jatropha curcas L. has been considered a potential source of seed oil for the production of biofuel. The plant ethnopharmacological applications are well known, but much of the information is empirical and lacking in scientific validation [ 2 ]. Terpenoid compounds are the major metabolites found in the Euphorbiaceae family. Among the terpenes, diterpenoids have dominated research in Jatropha species with respect to their novel chemical structures and medicinal values [ 3 ]. Recently, Oskoueian et al. [ 2 ] reported that extract of root and latex of J. curcas plant which contained phenolics, flavonoid and saponins showed notable antioxidant, anticancer and anti-inflammatory activities. These compounds have been reported to be involved in the biological activities of the plant [ 4 ].
Continuous efforts have been carried out to determine the presence of bioactive compounds in various plant materials, in particular, the agro-industrial by-products since they are renewable and abundantly available [ 4 ]. In the case of J. curcas kernel, the process of oil extraction produces a residue called kernel meal. This by-product has not been comprehensively studied, therefore limited information is available on the feasible applications of the meal as a potential source of bioactive compounds. Hence, the objective of the present study was to characterize the compounds present in the kernel meal and to investigate the biological activities of the meal extract. The information gathered would indicate the potential of the kernel meal as a source of bioactive compounds.
2. Results and Discussion
2.1. chemical composition.
The chemical composition of kernel meal obtained after oil extraction was determined. As shown in Table 1 , the meal is high in protein (61.8% w/w) but low in neutral detergent fiber (NDF) (9.7% w/w) and acid detergent fiber (ADF) (4.8% w/w). These results are comparable to those of J. curcas kernel meal from Mexico, India, Nicaragua and Cape Verde as reported by Makkar and Becker [ 1 ]. The phytochemical analyses of J. curcas kernel meal showed that total phenolics was 3.9 ± 0.23 mg tannic acid equivalents/g DM and total flavonoid was 0.4 ± 0.15 mg rutin equivalents/g DM ( Table 1 ). Total phenolic content is comparable to the value of 3.6 mg tannic acid equivalents/g reported by Makkar and Becker [ 1 ], while total flavonoid content of kernel meal has not been reported so far.
Chemical and phytochemical analyses of J. curcas kernel meal (on dry matter basis) * .
Neutral detergent fiber;
Acid detergent fiber;
Tannic acid equivalents;
Rutin equivalents;
Diosgenin equivalents;
Equivalent to phorbol 12-myristate,13 acetate;
Each value represents the mean ± S.E.M of three replicates.
Phenolic and flavonoid compounds occur ubiquitously in plants and a variety of biological activities have been attributed to them. These advantageous properties include antimicrobial, antiviral, anti-ulcerogenic, cytotoxic, anti-neoplastic, mutagenic, antioxidant, anti-hepatotoxic, anti-hypertensive, hypolipidemic, anti-platelet and anti-inflammatory activities. Many of these biological functions corresponded to their free radical scavenging and antioxidant activities [ 5 ].
Total saponin content was 19.0 ± 0.48 mg diosgenin equivalents/g DM. This value was lower when compared to kernel meal of J. curcas from Mexico, Cape Verde and India with the values of 28.5, 26.0 and 27.3 mg diosgenin equivalents/g DM, respectively [ 6 ]. Saponins are widely distributed amongst plants and it is believed that saponins naturally act to protect the plant against pathogens. Their main biological activities include haemolytic, molluscicidal, anti-inflammatory, antimicrobial, anti-parasitic, cytotoxic and anti-tumor [ 7 ]. The presence of both polar (sugar) and nonpolar (steroid or triterpene) groups provide saponins with strong surface-active properties that are responsible for many of their biological activities such as antioxidant, antimicrobial, anti-inflammatory and cytotoxicity [ 8 ].
Phorbol esters are esters of tetra-cyclic diterpenes which are widely distributed in plant species of the families Euporbiaceae and Thymelaceae and biological activities such as anti-HIV, anti-malaria and antimicrobial have been reported by Goel et al. [ 9 ]. The phorbol esters content of kernel meal was 3.0 ± 0.16 mg phorbol-12-myristate 13-acetate (PMA) equivalents/g DM. Phorbol esters are known to mimic the action of diacylglycerol to activate the protein kinase C, which regulates different signal transduction pathways and other cellular metabolic activities including proliferation, malignant transformation, differentiation and cell death [ 9 ]. The multiplicity of effects of phorbol esters on biological systems is associated with the presence of phorbol esters receptors [ 10 ] and the types of phorbol esters. Fujii et al. [ 10 ] and Park et al. [ 11 ] reported the induction of apoptosis using phorbol esters in prostate cancer cells and gastric cancer cells.
2.2. Phenolics and Flavonoids Analyses by HPLC
In this study, high performance liquid chromatography (HPLC) was used to determine the phenolic and flavonoid compounds in J. curcas kernel meal extracts. The analyses showed the presence of gallic acid and pyrogallol as phenolics ( Figure 1 ); rutin and myricetin as flavonoids ( Figure 2 ) and daidzein as isoflavonoid compound ( Figure 1 ) with the concentrations shown in Table 2 . Compounds that have been reported from J. curcas leaves including apigenin and its glycosides, vitexin and isovitexin, stigmasterol, β-sitosterol and gallic acid [ 12 ] while the root and stem contained gallic acid, ellagic acid, quercetin, coumaric acid, benzoic acid and salicylic acid [ 13 ].
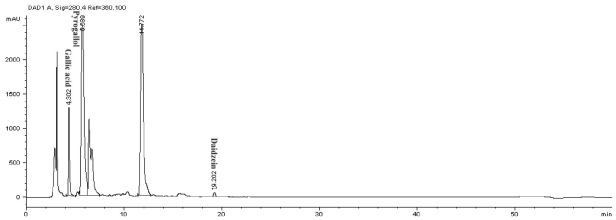
HPLC chromatogram of phenolic and isoflavonoid compounds from methanolic extract of J. curcas kernel meal detected at 280 nm.
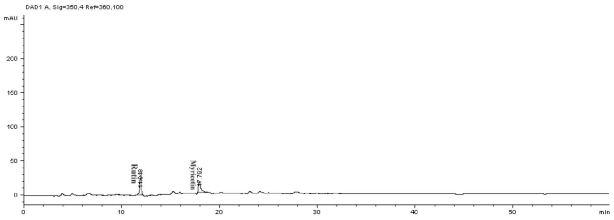
HPLC chromatogram of flavonoid compounds from methanolic extract of J. curcas kernel meal detected at 350 nm.
Concentrations of phenolic, flavonoid and isoflavonoid compounds in J. curcas kernel meal methanolic extract (μg/g) * .
All values are on DM basis;
2.3. Metabolite Composition Analysis by GC-MS
The GC-MS analysis is a rapid and simple method to determine the compounds present in the plant materials. Table 3 shows the major organic compounds detected by GC-MS in the methanolic and hot water extracts. More than half of the organic compounds in the methanolic extract consists of 2-(hydroxymethyl)-2 nitro-1,3-propanediol, β-sitosterol, 2-furancarboxaldehyde, 5-(hydroxymethy) and acetic acid. In the hot water extract, the most common compounds present are 2-furancarboxaldehyde, 5-(hydroxymethy), acetic acid and furfural (2-furancarboxaldehyde) adding up to about 60% of the total extracted materials. The 2-(Hydroxymethyl)-2 nitro-1,3-propanediol has been reported as microbicidal and it is used as a bacteriostat in disinfectants [ 14 ]. β-sitosterol, a plant sterol, shows antimicrobial [ 15 ], anti-inflammatory and cytotoxic activities [ 16 , 17 ]. The compound 2-furancarboxaldehyde, 5-(hydroxymethy) also shows antibacterial and antifungal activity applicable in pharmaceutical, cosmetics and pesticides [ 18 ]. Furfural is used as a flavor in foods, and in other products, such as cosmetics, fragrance, pesticide, herbicide, fungicide, insecticide and germicide [ 19 ]. According to Ryssel et al. [ 20 ], acetic acid also showed excellent bactericidal effects toward Proteus vulgaris , Pseudomonas aeruginosa and Acinetobacter baumannii . Therefore, the GC-MS results showed the presence of compounds with known activities indicating the J. curcas kernel meal as a potential source of functional metabolites.
Major organic compounds detected in the methanolic and hot water extracts of J. Curcas kernel meal.
2.4. Antibacterial Activity
Antibacterial activity of methanolic and hot water extracts of J. curcas kernel meal were tested against four Gram negative and four Gram positive pathogens at two concentrations (1.0 and 1.5 mg/disc). The results shown in Table 4 indicate significant difference ( P < 0.05) in inhibitory activity of methanolic and hot water extracts. The diameter of inhibition zones of methanolic extract ranged from 0.35 to 1.25 cm while for hot water extract ranged from 0.00 to 0.75 cm at 1.0 mg/disc. Antimicrobial activity of the methanolic extract appeared to be more effective than water extract, since methanol could extract a wide variety of active components compared to hot water. The size of inhibition zones increased with 1.5 mg/disc. At this concentration, the antibacterial effect of methanolic extract was similar for both Gram negative and Gram positive bacteria, except for P. aeruginosa , which showed moderate zone of inhibition. This Gram negative bacteria seemed to be less sensitive to other plant extracts [ 21 ].
Inhibition zones of J. curcas kernel meal extracts against pathogenic bacteria at the concentration of 1.0 and 1.5 mg/disc.
Means with different superscripts within rows are significantly different ( P < 0.05); Analyses were done in triplicate;
S.E.M: Standard error of mean.
Escherichia coli seemed to be the most susceptible bacterial species to methanolic and hot water extracts at 1.0 and 1.5 mg/disc extract concentration. The antibacterial activities of both kanamycin and streptomycin were as expected and some of the clearing zones of pathogens were comparable to that of antibiotics, particularly when 1.5 mg/disc extracts were tested.
Various reports have shown the antimicrobial activity of extracts from different parts of J. curcas plant. However, the efficacy of extracts to inhibit microbial growth seemed to vary according to extraction procedures and assay methods. Igbinosa et al. [ 22 ] have reported the in vitro antimicrobial activity of crude methanolic and water extracts of the J. curcas stem bark against a wide spectrum of microorganisms with zones of inhibition ranging from 0.8 to 2 and 0 to 0.8 cm for methanol and water extracts at the concentration of 1.0 mg per well (0.6 mm) respectively. In another study, Atindehou et al. [ 23 ] reported the antimicrobial activity of the leaves and roots of J. curcas against E. coli , P. aeruginosa , S. aureus and E. faecalis .
Total phenolics generally possess antimicrobial activities which provide chemical barriers for invading microorganisms. On the other hand, flavonoids inhibit bacterial growth by inhibition of DNA gyrase, cytoplasmic membrane function and energy metabolism [ 24 ]. Gallic acid and pyrogallol as phenolic compounds, daidzein as isoflavonoid and rutin and myricetin as flavonoids ( Table 2 ) are substantially effective as antibacterial agent [ 24 ]. Additionally, the saponins present in J. curcas kernel meal could also contributed to the antimicrobial activity as saponins have been shown to possess antibacterial activity against several Gram positive and Gram negative bacteria [ 7 ]. Furthermore, phorbol esters ( Table 1 ) could also act as antimicrobial agent as this property has been demonstrated by Chumkaew et al. [ 25 ].
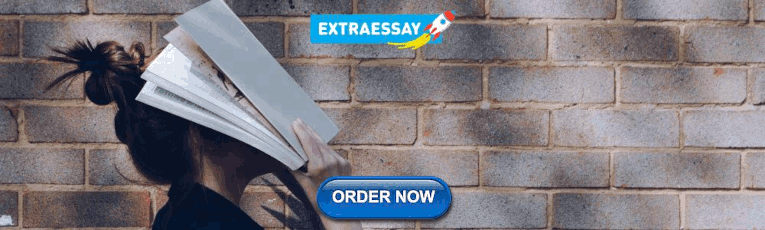
2.5. Antioxidant Activity
The results shown in Figure 3 indicate the 2,2-diphenyl-1-picrylhydrazyl (DPPH) radical scavenging activity of J. curcas kernel meal extracts at different concentrations. The inhibition of DPPH radical scavenging activity increased in a dose-dependent manner. Table 5 shows the IC 50 (required concentration to scavenge 50% of DPPH radicals) concentration of extracts. Free radical scavenging activity of methanolic extract showed a value of 1.6 mg/mL but hot water extract did not show an IC 50 value, even at the highest concentration used in this study. Methanolic extract of J. curcas kernel meal appeared to be more active as compared to the hot water extract in scavenging the free radicals. Reference antioxidants vitamin C, BHT and β-carotene showed IC 50 values of 0.3, 0.3 and 1.5 mg/mL respectively. Both extracts showed lower activities than vitamin C and BHT due to the significantly ( p < 0.05) higher IC 50 values required to inhibit the free radicals ( Table 5 ). The methanolic extract activity was similar to β-carotene as no significant difference was observed between their IC 50 values ( Table 5 ). It has been reported that the strong free radical scavenging activities in latex, root and stem of J. curcas was attributed to the presence of phenolic compounds [ 2 , 13 ].
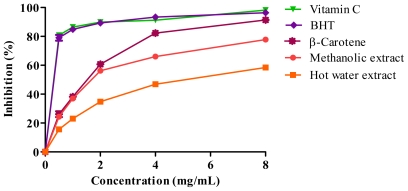
The free radical scavenging activity of J. curcas kernel meal extracts and reference antioxidants.
The IC 50 values of J. curcas kernel meal extracts and reference antioxidants.
Values with different subscripts in each column are significantly different ( p < 0.05); Analyses were done in triplicate;
Ferric reducing antioxidant power (FRAP) of extracts and reference antioxidants (vitamin C, BHT and β-carotene) is presented in Figure 4 . Reference antioxidants showed higher reducing power followed by methanolic and hot water extract. The IC 50 value (the concentration to reduce 50% of Fe 3+ to Fe 2+ as an index for antioxidant potential) in FRAP for vitamin C, BHT and β-carotene were 0.3, 0.3 and 2.6 mg/mL, respectively ( Table 5 ), while for the methanolic and hot water extracts were 3.0 and 8.0 mg/mL, respectively. As observed in the DPPH assay, FRAP assay also showed similar IC 50 values between methanolic extract and β-carotene ( Table 5 ).
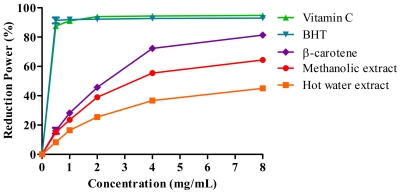
Ferric reducing activity of J. curcas kernel meal extracts and reference antioxidants.
The results indicate that the extracts were more active in scavenging free radicals compared to ferric reducing power activity. In FRAP assay, the general ability of the extracts to donate electrons is tested whereas, in the DPPH assay, reduction by hydrogen atoms are also involved. Hence, the free radical scavenging activity assay was a better indicator of antioxidant activity of kernel meal than FRAP based on the reaction mechanisms proposed. In complex systems, such as food, various different mechanisms may contribute to oxidative processes. Therefore, it is important to characterize the extracts by a variety of antioxidant assays [ 26 ].
The presence of phenolics (gallic acid, pyrogallol), flavonoids (rutin, myricetin) and isoflavonoid (daidzein) in the kernel meal ( Table 2 ) may contribute to the antioxidant activity of extracts. These compounds have been identified as some of the major compounds involved in antioxidant activity [ 27 ]. However, saponins in the kernel meal ( Table 1 ) might be also involved, because Sparg et al. [ 7 ] reported the antioxidant activity of these compounds.
2.6. Cytotoxicity Activity
Phytochemical analyses, antimicrobial and antioxidant activities showed that the methanolic extract was more active compared to hot water extract. Therefore, cytotoxicity assay was performed using the methanolic extract. It is known that different cell lines might exhibit different sensitivities while treated with the same plant extract, therefore screening plant crude extract against various cell lines is a first step in determining the most susceptible cancer cells. Apart from that, breast and cervical cancers are very common manifestations, hence the MCF-7 and HeLa cell lines were used to investigate the effects of the plant extract. Chang liver cells (human hepatocytes) were also used to determine the hepatoxicity of extract used in this study. Cytotoxicity assay results demonstrated that the methanolic extract of J. curcas kernel meal inhibited the growth and proliferation of MCF-7, HeLa and Chang liver cells in a dose-dependent manner ( Figure 5 ). Methanolic extract at the concentration of 6.25 μg/mL and above decreased the cell viabilities significantly ( P < 0.0001) in all cell lines used in this study as compared to the untreated control.
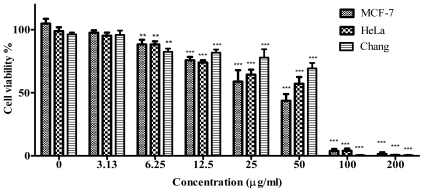
Effect of methanolic extract of J. curcas kernel meal on MCF-7, HeLa and Chang liver cell viabilities. All bars represent the mean ± standard error of three independent experiments. *** P < 0.0001 and ** P < 0.001 indicates significant difference compared to the untreated control group.
In this cytotoxicity activity study, Tamoxifen was used as a positive control. As shown in Figure 6 , Tamoxifen also inhibited the growth and proliferation of MCF-7, HeLa and Chang liver cells in a dose dependent manner. At 6.25 μg/mL and above, cell viabilities decreased significantly ( P < 0.0001) in all cell lines when compared to the untreated control.
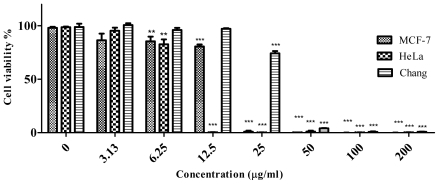
Effect of Tamoxifen as a positive control on MCF-7, HeLa and Chang liver cell viabilities. All bars represent the mean ± standard error of three independent experiments. *** P < 0.0001 and ** P < 0.001 indicates significant difference compared to the untreated control group.
The CC 50 values of methanolic extract and Tamoxifen are presented in Table 6 . The MCF-7 cells were found to be more susceptible to the methanolic extract as compared to the HeLa and Chang cells. Most anticancer drugs have been discovered through random screening of plant materials. The National Cancer Institute requires a CC 50 value of less than 30 μg/mL for the active crude extracts against cancer cells [ 28 ]. Since the methanolic extract showed CC 50 values less than National Cancer Institute requirement toward MCF-7 cells, methanolic extract could be a promising source of bioactive compounds that could be purified for the development of anticancer drugs in order to treat breast cancer.
The CC 50 values of extracts and positive control (Tamoxifen) against MCF-7, HeLa and Chang liver cell lines.
Analyses were done in triplicate;
The CC 50 concentrations of Tamoxifen ( Table 6 ) for MCF-7, HeLa and Chang liver cell lines were 17.3, 8.89, 33.6 μg/mL, respectively. The activity of Tamoxifen toward MCF-7, HeLa and Chang were significantly ( p < 0.0001) higher than methanolic extract. However, normal Chang liver cells were also susceptible to both methanolic extract and Tamoxifen. It is interesting to note that the CC 50 concentration of Tamoxifen was about half of that methanolic extract. At present it is not known which particular compound(s) present in the kernel meal methanolic extract exert the cytotoxicity effect in both cancer and, to some extent, Chang liver cells.
The presence of bioactive compounds in other J. curcas plant parts has been reported recently. Oskoueian et al. [ 2 ] demonstrated the cytotoxic effect of J. curcas root and latex methanolic extract with CC 50 values of 18.3 ± 0.98 and 70.1 ± 2.06 μg/mL against HT-29 cloned cancer cells, respectively; and 33.3 ± 0.75 and 60.1 ± 0.89 μg/mL toward Chang liver cells, respectively. Besides root and latex, leaves methanolic extract was also observed to be effective against B16F10 melanoma cells with CC 50 value of 24.8 μg/mL [ 29 ]. Moreover, Fujii et al. [ 10 ] reported that PMA induced apoptosis in prostate cancer cells through overexpression of PKCδ. Park et al. [ 11 ] also concluded that the PMA induce apoptosis in gastric cancer cells through activation of PKC and the activation of serine protease(s) and caspase-3/CPP32. All these results indicate the potential of J. curcas plant as a source of bioactive compounds, but, the exact nature of the metabolite involved has to be elucidated, although it has been reported that phenolics, flavonoids, saponins and phorbol esters have been shown to be cytotoxic on different cell lines [ 9 ].
3. Experimental Section
3.1. sample and extract preparation.
The Jatropha curcas L. plant was collected from the farm of Faculty of Agriculture, Universiti Putra Malaysia with the GPS location of 3°0′26.91″N latitude and 101°42′13.24″E longitude for identification by Mr. Shamsul Khamis. A voucher specimen (SK1764/2010) was deposited in the Phytomedicinal Herbarium, Institute of Bioscience, Universiti Putra Malaysia, Serdang, Selangor, Malaysia. Upon confirmation of the plant, the ripe J. curcas seeds were collected from the farm, air dried, dehulled and kept at 4 °C for further analysis. The kernels isolated were ground, using a mechanical grinder and oil was extracted by Soxhlet apparatus, using petroleum ether (boiling point of 40–60 °C) for 16 h [ 30 ]. Methanolic extract of J. curcas kernel meal was prepared according to Crozier et al. [ 27 ]. Hot water extract was prepared as described by Gulcin et al. [ 31 ]. The extracts were filtered through Whatman No.1 paper and evaporated by using vacuum rotary evaporator (Buchii, Switzerland). The crude extracts were re-dissolved in methanol for antioxidant, antimicrobial and HPLC analyses, or in dimethyl sulfoxide for cytotoxicity assay. The extractions were repeated three times.
3.2. Chemical Constituents
Chemical constituent analyses were conducted according to AOAC [ 32 ]. The fiber fractions which consisted of neutral detergent fiber (NDF) and acid detergent fiber (ADF) were determined according to Van Soest et al. [ 33 ]. The analyses were repeated three times.
3.3. Phytochemical Analyses
3.3.1. total phenolics.
Total phenolics was determined by using Folin–Ciocalteu reagent according to Ismail et al. [ 26 ] and total phenolic results were expressed as mg tannic acid equivalentss/g DM of the kernel meal. The analysis was conducted in triplicate.
3.3.2. Total Flavonoids
Total flavonoids was determined based on aluminium chloride colorimetric assay described by Ismail et al. [ 26 ]. Total flavonoid compound of extracts was expressed as mg rutin equivalents/g DM of the kernel meal. The analysis was conducted in triplicate.
3.3.3. Total Saponins
Total saponin was determined according to Makkar et al. [ 8 ] based on vanillin-sulfuric acid colorimetric reaction and results expressed as mg diosgenin equivalents/g DM of the kernel meal. The analysis was conducted in triplicate.
3.4. Analyses of Phenolic and Flavonoid Compounds by HPLC
The phenolic and flavonoid compounds of J. curcas kernel meal were quantitatively measured by HPLC based on the method described by Crozier et al. [ 27 ] with some modification. Phenolic standards were gallic acid, syringic acid, vanillic acid, salicylic acid, caffeic acid and pyrogallol. Flavonoid standards used in this research were quercetin, rutin, myricetin, kaempferol, naringin and apigenin and isoflavonoid standards were genistein and daidzein. An aliquot of sample extracts was loaded on the HPLC equipped with an analytical column Intersil ODS-3 (5 μm 4.6 × 150 mm, Gl Science Inc.). Solvents comprising deionized water (solvent A) and acetonitrile (solvent B) were used. The pH of water was adjusted to 2.5 with trifluoroacetic acid. The phenolic and isoflavonoid compounds were detected at 280 nm while flavonoid compounds at 350 nm. The column was equilibrated by 85% solvent A and 15% solvent B. Then the ratio of solvent B was increased to 85% in 50 min followed by reducing solvent B to 15% in 55 min. This ratio was maintained for 60 min for each sample analysis with flow rate at 0.6 mL/min. The analysis was repeated three times.
3.5. Analysis of Phorbol Esters
Phorbol esters were determined based on Makkar et al. [ 8 ]. An aliquot of sample extracts was loaded on a high-performance liquid chromatography (HPLC Agilent-1200) series instrument equipped with a UV-Vis photodiode array (DAD) detector, binary pump, vacuum degasser, auto sampler and analytical column Agilent Reverse-phase C 18 LiChrospher 100 (250 × 4 mm I.D and 5 μm pore size). The solvents were deionized water (solvent A) and acetonitrile (solvent B). The absorbance was read at 280 nm and results were expressed as equivalents to phorbol-12-myristate 13-acetate standard. The analysis was done in triplicate.
3.6. Analysis of Metabolites by Gas Chromatography-Mass Spectrometry (GC-MS)
The chemical composition of the extracts was characterized by GC-MS based on the method described by Hossain and Rahman [ 34 ]. The GC-MS used was a Schimadzu QP2010PLUS system. Six μL were analysed on a BPX-5 SGE ultra-low-bleed 5% phenyl polydimethylsiloxane capillary column (30 m × 0.25 mm i.d. × 0.25 μm film thickness). Splitless injection was performed with a purge time of 1.0 min. The carrier gas was helium at a flow rate of 1 mL min −1 . The column temperature was maintained at 50 °C for 3 min, then programmed at 5°C min −1 to 80 °C and then at 10 °C min −1 to 340 °C. The inlet and detector temperature was 250 °C and 340 °C, respectively, and the solvent delay was 4 min. The identification of the peaks was based on computer matching of the mass spectra with the National Institute of Standards and Technology (NIST 08 and NIST 08s) library and by direct comparison with published data. The analyses were carried out in triplicate.
3.7. Antibacterial Activity
The antibacterial assay of J. curcas kernel meal extracts was carried out by the disc diffusion method as described by Boussaada et al. [ 21 ] against Gram positive ( Micrococcus luteus , Bacillus subtilis B145, Bacillus cereus B43 and Staphylococcus aureus S1431) and Gram negative ( Enterobacter aerogenes , Klebsiella pneumonia K36, Escherichia coli E256 and Pseudomonas aeruginosa PI96) pathogens. All the bacteria were purchased from the Institute of Malaysian Research (IMR) and maintained in the Department of Microbiology, Faculty of Biotechnology and Biomolecular Sciences, Universiti Putra Malaysia. The positive control without extracts (solvent) and reference controls using two standard antibiotics (kanamycin and streptomycin) were included in the assay. The extracts inhibitions were corrected based on positive control and compared with those of reference control. The experiments were conducted in triplicate.
3.8. Antioxidant Activity
3.8.1. dpph radical-scavenging activity.
Free radical scavenging activity of extracts was determined with 1,1-diphenyl-2-picryl-hydrazil (DPPH) as free radicals according to Ismail et al. [ 26 ]. The absorbance was measured at 515 nm by using a spectrophotometer. Vitamin C, butylated hydroxytoluene (BHT) and β-carotene were used as standard antioxidants. The assay was performed in triplicate.
3.8.2. Ferric-Reducing Antioxidant Power (FRAP) Assay
The ferric reducing antioxidant power (FRAP) of the extracts was determined as described by Yen and Chen [ 35 ]. Vitamin C, butylated hydroxytoluene (BHT) and β-carotene were used as standard antioxidants. The assay was performed in triplicate.
3.9. Cytotoxicity Assay
Two cancer cell line, including human breast cancer cell line (MCF-7 ATCC: HTB-22) and human cervical adenocarcinoma (HeLa ATCC: CCL-2) and one normal cell line, human hepatocytes (Chang ATCC: CCL-13) were purchased from the American Type Culture Collection (ATCC). Cell lines were grown as monolayers in a T-75 cm 2 culture flask. The Dulbecco’s Modified Eagle Medium (DMEM) was supplemented with 2.0 g/L sodium bicarbonate and 10% fetal bovine serum. The cultured cell lines were maintained in a humidified atmosphere of 5% CO 2 at 37 °C and were harvested when they reached 80% confluency for the cytotoxic test. Cytotoxicity of J. curcas kernel meal extract was measured by MTT cell proliferation assay [ 36 ]. Cytotoxic concentration was expressed as CC 50 (concentration of the sample that decreased the number of viable cells to 50% of the control cell) which was calculated by Graph Pad Prism 5 software. All determinations were performed in triplicate.
3.10. Statistical Analyses
Inhibition zones of antibacterial assay subjected to the general linear models procedure of SAS [ 37 ] in a completely randomized design and the means were compared with Duncan’s Multiple Range test. The difference was considered significant when the P value was <0.05. GraphPad Prism 5 software (GraphPad Software Inc., San Diego, CA) was used for all the statistical analyses in cytotoxic assay.
4. Conclusions
The kernel meal obtained from J. curcas seeds grown in Malaysia contains high percentage of crude protein and relatively low fiber. Analysis by HPLC showed the presence of various phenolics and flavonoids, together with saponins and phorbol esters, while GC-MS analyses indicated the presence of other metabolites that are probably involved in the antibacterial, antioxidant and cytotoxicity activities observed in this study. The ability of methanolic extract to inhibit breast cancer cell growth indicates the potential value of J. curcas kernel meal as an alternative source of therapeutic agents which requires further investigation.
Acknowledgments
The authors would like to thank the Ministry of Higher Education of Malaysia for the grant provided under the Fundamental Research Grant Scheme (Project No. 01-11-08-660FR).
- 1. Makkar H, Becker K. Jatropha curcas, a promising crop for the generation of biodiesel and value-added coproducts. Eur J Lipid Sci Technol. 2009;111:773–787. [ Google Scholar ]
- 2. Oskoueian E, Abdullah N, Saad WZ, Omar AR, Ahmad S, Kuan WB, Zolkifli NA, Hendra R, Ho YW. Antioxidant, anti-inflammatory and anticancer activities of methanolic extracts from Jatropha curcas Linn. J Med Plants Res. 2011;5:49–57. [ Google Scholar ]
- 3. Devappa RK, Makkar HPS, Becker K. Jatropha Diterpenes: a Review. J Am Oil Chem Soc. 2011;88:301–322. [ Google Scholar ]
- 4. Balasundram N, Sundram K, Samman S. Phenolic compounds in plants and agri-industrial by-products: Antioxidant activity, occurrence, and potential uses. Food Chem. 2006;99:191–203. [ Google Scholar ]
- 5. Muraoka S, Miura T. Inhibition of xanthine oxidase by phytic acid and its antioxidative action. Life Sci. 2004;74:1691–1700. doi: 10.1016/j.lfs.2003.09.040. [ DOI ] [ PubMed ] [ Google Scholar ]
- 6. Devappa RK, Swamylingappa B. Biochemical and nutritional evaluation of Jatropha protein isolate prepared by steam injection heating for reduction of toxic and anti nutritional factors. J Sci Food Agric. 2008;88:911–919. [ Google Scholar ]
- 7. Sparg SG, Light ME, Van Staden J. Biological activities and distribution of plant saponins. J Ethnopharmacol. 2004;94:219–243. doi: 10.1016/j.jep.2004.05.016. [ DOI ] [ PubMed ] [ Google Scholar ]
- 8. Makkar HPS, Siddhuraju P, Becker K. Methods in Molecular Biology: Plant Secondary Metabolites. Humana Press; Totowa, NJ, USA: 2007. pp. 93–100. [ DOI ] [ PubMed ] [ Google Scholar ]
- 9. Goel G, Makkar HPS, Francis G, Becker K. Phorbol esters: structure, biological activity, and toxicity in animals. Int J Toxicol. 2007;26:279–288. doi: 10.1080/10915810701464641. [ DOI ] [ PubMed ] [ Google Scholar ]
- 10. Fujii T, Garcia-Bermejo ML, Bernabo JL, Caamano J, Ohba M, Kuroki T, Li L, Yuspa SH, Kazanietz MG. Involvement of protein kinase C (PKC) in phorbol ester-induced apoptosis in LNCaP prostate cancer cells. J Biol Chem. 2000;275:7574–7582. doi: 10.1074/jbc.275.11.7574. [ DOI ] [ PubMed ] [ Google Scholar ]
- 11. Park IC, Park MJ, Rhee CH, Lee JI, Choe TB, Jang JJ, Lee SH, Hong SI. Protein kinase C activation by PMA rapidly induces apoptosis through caspase-3/CPP32 and serine protease(s) in a gastric cancer cell line. Int J Oncol. 2001;18:1077–1083. doi: 10.3892/ijo.18.5.1077. [ DOI ] [ PubMed ] [ Google Scholar ]
- 12. Chhabra SC, Mahunnah RLA, Mshiu EN. Plants used in traditional medicine in Eastern Tanzania. III. Angiosperms (Euphorbiaceae to Menispermaceae) J Ethnopharmacol. 1990;28:255–283. doi: 10.1016/0378-8741(90)90078-8. [ DOI ] [ PubMed ] [ Google Scholar ]
- 13. El Diwani G, El Rafie S, Hawash S. Antioxidant activity of extracts obtained from residues of nodes leaves stem and root of Egyptian Jatropha curcas. Afr J Pharm Pharacol. 2009;3:521–530. [ Google Scholar ]
- 14. Popendorf W, Selim M, Lewis MQ. Exposure while applying industrial antimicrobial pesticides. Am Ind Hyg Assoc J. 1995;56:993–1001. doi: 10.1080/15428119591016421. [ DOI ] [ PubMed ] [ Google Scholar ]
- 15. Ajaiyeoba EO, Onocha PA, Nwozo SO, Sama W. Antimicrobial and cytotoxicity evaluation of Buchholzia coriacea stem bark. Fitoterapia. 2003;74:706–709. doi: 10.1016/s0367-326x(03)00142-4. [ DOI ] [ PubMed ] [ Google Scholar ]
- 16. Gupta MB, Nath R, Srivastava N, Shanker K, Kishor K, Bhargava KP. Anti-inflammatory and antipyretic activities of β-sitosterol. Planta Med. 1980;39:157–163. doi: 10.1055/s-2008-1074919. [ DOI ] [ PubMed ] [ Google Scholar ]
- 17. Awad A, Chen YC, Fink C, Hennessey T. Sitosterol inhibits HT-29 human colon cancer cell growth and alters membrane lipids. Anticancer Res. 1996;16:2797–2804. [ PubMed ] [ Google Scholar ]
- 18. Rigal L, Gaset A. Direct preparation of 5-hydroxymethyl-2-furancarboxaldehyde from polyholosides: a chemical valorisation of the Jerusalem artichoke (Helianthus tuberosus L.) Biomass. 1983;3:151–163. [ Google Scholar ]
- 19. Morales FJ. Hydroxymethylfurfural (HMF) and Related Compounds. In: Stadler RH, Lineback DR, editors. Process-Induced Food Toxicants. John Wiley & Sons, Inc; Hoboken, NJ, USA: 2008. pp. 135–174. [ Google Scholar ]
- 20. Ryssel H, Kloeters O, Germann G, Schafer T, Wiedemann G, Oehlbauer M. The antimicrobial effect of acetic acid-An alternative to common local antiseptics? Burns. 2009;35:695–700. doi: 10.1016/j.burns.2008.11.009. [ DOI ] [ PubMed ] [ Google Scholar ]
- 21. Boussaada O, Chriaa J, Nabli R, Ammar S, Saidana D, Mahjoub M, Chraeif I, Helal A, Mighri Z. Antimicrobial and antioxidant activities of methanol extracts of Evax pygmaea (Asteraceae) growing wild in Tunisia. World J Microb Biot. 2008;24:1289–1296. [ Google Scholar ]
- 22. Igbinosa O, Igbinosa E, Aiyegoro O. Antimicrobial activity and phytochemical screening of stem bark extracts from Jatropha curcas (Linn) Afr J Pharm Pharacol. 2009;3:58–62. [ Google Scholar ]
- 23. Atindehou K, Kone M, Terreaux C, Traore D, Hostettmann K, Dosso M. Evaluation of the antimicrobial potential of medicinal plants from the Ivory Coast. Phytother Res. 2002;16:497–502. doi: 10.1002/ptr.970. [ DOI ] [ PubMed ] [ Google Scholar ]
- 24. Cushnie T, Lamb A. Antimicrobial activity of flavonoids. Int J Antimicro Ag. 2005;26:343–356. doi: 10.1016/j.ijantimicag.2005.09.002. [ DOI ] [ PMC free article ] [ PubMed ] [ Google Scholar ]
- 25. Chumkaew P, Karalai C, Ponglimanont C, Chantrapromma K. Antimycobacterial activity of phorbol esters from the fruits of Sapium indicum. J Nat Prod. 2003;66:540–543. doi: 10.1021/np0204489. [ DOI ] [ PubMed ] [ Google Scholar ]
- 26. Ismail HI, Chan KW, Mariod AA, Ismail M. Phenolic content and antioxidant activity of cantaloupe (cucumis melo) methanolic extracts. Food Chem. 2010;119:643–647. [ Google Scholar ]
- 27. Crozier A, Lean M, McDonald M, Black C. Quantitative analysis of the flavonoid content of commercial tomatoes, onions, lettuce, and celery. J Agric Food Chem. 1997;45:590–595. [ Google Scholar ]
- 28. Boik JC. Natural Compounds in Cancer Therapy. Oregon Medical Press; Princeton, MN, USA: 2001. pp. 149–190. [ Google Scholar ]
- 29. Balaji R, Rekha N, Deecaraman M, Manikandan L. Antimetastatic and antiproliferative activity of methanolic fraction of Jatropha curcas against B16F10 melanoma induced lung metastasis in C57BL/6 mice. Afr J Pharm Pharacol. 2009;3:547–555. [ Google Scholar ]
- 30. Martinez-Herrera J, Siddhuraju P, Francis G, Davila-Ortiz G, Becker K. Chemical composition, toxic/antimetabolic constituents, and effects of different treatments on their levels, in four provenances of Jatropha curcas L. from Mexico. J Food Chem. 2006;96:80–89. [ Google Scholar ]
- 31. Gulcin I, Gungor Sat I, Beydemir S, Elmastas M, Irfan Kufrevioglu O. Comparison of antioxidant activity of clove (Eugenia caryophylata Thunb) buds and lavender (Lavandula stoechas L.) Food Chem. 2004;87:393–400. [ Google Scholar ]
- 32. AOAC. Official Methods of Analysis. 15th ed. Association of Official Analytical Chemists; Washington, DC, USA: 1990. pp. 64–87. [ Google Scholar ]
- 33. Van Soest PJ, Robertson JB, Lewis BA. Methods for dietary fiber, neutral detergent fiber, and nonstarch polysaccharides in relation to animal nutrition. J Dairy Sci. 1991;74:35–83. doi: 10.3168/jds.S0022-0302(91)78551-2. [ DOI ] [ PubMed ] [ Google Scholar ]
- 34. Hossain M, Rahman A. Chemical composition of bioactive compounds by GC-MS screening and anti-fungal properties of the crude extracts of cabbage samples. Asian J Biotechnol. 2011;3:68–76. [ Google Scholar ]
- 35. Yen G, Chen H. Antioxidant activity of various tea extracts in relation to their antimutagenicity. J Agric Food Chem. 1995;43:27–32. [ Google Scholar ]
- 36. Sharif R, Ghazali A, Rajab N, Haron H, Osman F. Toxicological evaluation of some Malaysian locally processed raw food products. Food Chem Toxicol. 2008;46:368–374. doi: 10.1016/j.fct.2007.08.010. [ DOI ] [ PubMed ] [ Google Scholar ]
- 37. SAS. SAS, Statistical Analysis Institute (Version 9.1.3) SAS Institute Inc; Cary, NC, USA: 2003. [ Google Scholar ]
- View on publisher site
- PDF (310.1 KB)
- Collections
Similar articles
Cited by other articles, links to ncbi databases.
- Download .nbib .nbib
- Format: AMA APA MLA NLM
Add to Collections
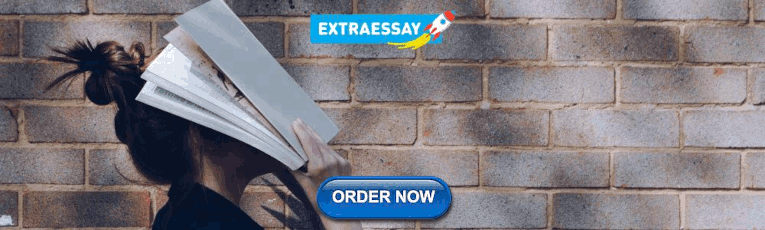
IMAGES
VIDEO