- PRO Courses Guides New Tech Help Pro Expert Videos About wikiHow Pro Upgrade Sign In
- EDIT Edit this Article
- EXPLORE Tech Help Pro About Us Random Article Quizzes Request a New Article Community Dashboard This Or That Game Forums Popular Categories Arts and Entertainment Artwork Books Movies Computers and Electronics Computers Phone Skills Technology Hacks Health Men's Health Mental Health Women's Health Relationships Dating Love Relationship Issues Hobbies and Crafts Crafts Drawing Games Education & Communication Communication Skills Personal Development Studying Personal Care and Style Fashion Hair Care Personal Hygiene Youth Personal Care School Stuff Dating All Categories Arts and Entertainment Finance and Business Home and Garden Relationship Quizzes Cars & Other Vehicles Food and Entertaining Personal Care and Style Sports and Fitness Computers and Electronics Health Pets and Animals Travel Education & Communication Hobbies and Crafts Philosophy and Religion Work World Family Life Holidays and Traditions Relationships Youth
- Browse Articles
- Learn Something New
- Quizzes Hot
- Happiness Hub
- This Or That Game
- Train Your Brain
- Explore More
- Support wikiHow
- About wikiHow
- Log in / Sign up
- Education and Communications
- College University and Postgraduate
- Academic Writing
- Research Papers
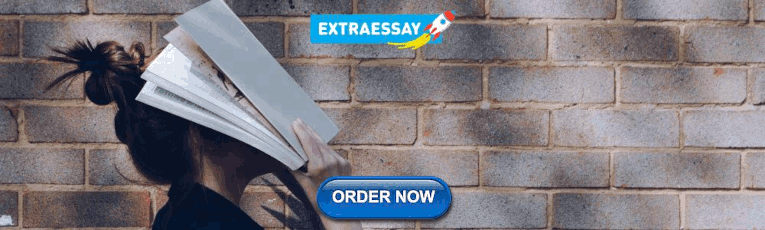
How to Write and Publish Your Research in a Journal
Last Updated: May 26, 2024 Fact Checked
Choosing a Journal
Writing the research paper, editing & revising your paper, submitting your paper, navigating the peer review process, research paper help.
This article was co-authored by Matthew Snipp, PhD and by wikiHow staff writer, Cheyenne Main . C. Matthew Snipp is the Burnet C. and Mildred Finley Wohlford Professor of Humanities and Sciences in the Department of Sociology at Stanford University. He is also the Director for the Institute for Research in the Social Science’s Secure Data Center. He has been a Research Fellow at the U.S. Bureau of the Census and a Fellow at the Center for Advanced Study in the Behavioral Sciences. He has published 3 books and over 70 articles and book chapters on demography, economic development, poverty and unemployment. He is also currently serving on the National Institute of Child Health and Development’s Population Science Subcommittee. He holds a Ph.D. in Sociology from the University of Wisconsin—Madison. There are 13 references cited in this article, which can be found at the bottom of the page. This article has been fact-checked, ensuring the accuracy of any cited facts and confirming the authority of its sources. This article has been viewed 708,870 times.
Publishing a research paper in a peer-reviewed journal allows you to network with other scholars, get your name and work into circulation, and further refine your ideas and research. Before submitting your paper, make sure it reflects all the work you’ve done and have several people read over it and make comments. Keep reading to learn how you can choose a journal, prepare your work for publication, submit it, and revise it after you get a response back.
Things You Should Know
- Create a list of journals you’d like to publish your work in and choose one that best aligns with your topic and your desired audience.
- Prepare your manuscript using the journal’s requirements and ask at least 2 professors or supervisors to review your paper.
- Write a cover letter that “sells” your manuscript, says how your research adds to your field and explains why you chose the specific journal you’re submitting to.

- Ask your professors or supervisors for well-respected journals that they’ve had good experiences publishing with and that they read regularly.
- Many journals also only accept specific formats, so by choosing a journal before you start, you can write your article to their specifications and increase your chances of being accepted.
- If you’ve already written a paper you’d like to publish, consider whether your research directly relates to a hot topic or area of research in the journals you’re looking into.

- Review the journal’s peer review policies and submission process to see if you’re comfortable creating or adjusting your work according to their standards.
- Open-access journals can increase your readership because anyone can access them.

- Scientific research papers: Instead of a “thesis,” you might write a “research objective” instead. This is where you state the purpose of your research.
- “This paper explores how George Washington’s experiences as a young officer may have shaped his views during difficult circumstances as a commanding officer.”
- “This paper contends that George Washington’s experiences as a young officer on the 1750s Pennsylvania frontier directly impacted his relationship with his Continental Army troops during the harsh winter at Valley Forge.”

- Scientific research papers: Include a “materials and methods” section with the step-by-step process you followed and the materials you used. [5] X Research source
- Read other research papers in your field to see how they’re written. Their format, writing style, subject matter, and vocabulary can help guide your own paper. [6] X Research source

- If you’re writing about George Washington’s experiences as a young officer, you might emphasize how this research changes our perspective of the first president of the U.S.
- Link this section to your thesis or research objective.
- If you’re writing a paper about ADHD, you might discuss other applications for your research.

- Scientific research papers: You might include your research and/or analytical methods, your main findings or results, and the significance or implications of your research.
- Try to get as many people as you can to read over your abstract and provide feedback before you submit your paper to a journal.

- They might also provide templates to help you structure your manuscript according to their specific guidelines. [11] X Research source

- Not all journal reviewers will be experts on your specific topic, so a non-expert “outsider’s perspective” can be valuable.

- If you have a paper on the purification of wastewater with fungi, you might use both the words “fungi” and “mushrooms.”
- Use software like iThenticate, Turnitin, or PlagScan to check for similarities between the submitted article and published material available online. [15] X Research source

- Header: Address the editor who will be reviewing your manuscript by their name, include the date of submission, and the journal you are submitting to.
- First paragraph: Include the title of your manuscript, the type of paper it is (like review, research, or case study), and the research question you wanted to answer and why.
- Second paragraph: Explain what was done in your research, your main findings, and why they are significant to your field.
- Third paragraph: Explain why the journal’s readers would be interested in your work and why your results are important to your field.
- Conclusion: State the author(s) and any journal requirements that your work complies with (like ethical standards”).
- “We confirm that this manuscript has not been published elsewhere and is not under consideration by another journal.”
- “All authors have approved the manuscript and agree with its submission to [insert the name of the target journal].”

- Submit your article to only one journal at a time.
- When submitting online, use your university email account. This connects you with a scholarly institution, which can add credibility to your work.

- Accept: Only minor adjustments are needed, based on the provided feedback by the reviewers. A first submission will rarely be accepted without any changes needed.
- Revise and Resubmit: Changes are needed before publication can be considered, but the journal is still very interested in your work.
- Reject and Resubmit: Extensive revisions are needed. Your work may not be acceptable for this journal, but they might also accept it if significant changes are made.
- Reject: The paper isn’t and won’t be suitable for this publication, but that doesn’t mean it might not work for another journal.

- Try organizing the reviewer comments by how easy it is to address them. That way, you can break your revisions down into more manageable parts.
- If you disagree with a comment made by a reviewer, try to provide an evidence-based explanation when you resubmit your paper.

- If you’re resubmitting your paper to the same journal, include a point-by-point response paper that talks about how you addressed all of the reviewers’ comments in your revision. [22] X Research source
- If you’re not sure which journal to submit to next, you might be able to ask the journal editor which publications they recommend.

Expert Q&A
You might also like.

- If reviewers suspect that your submitted manuscript plagiarizes another work, they may refer to a Committee on Publication Ethics (COPE) flowchart to see how to move forward. [23] X Research source Thanks Helpful 0 Not Helpful 0

- ↑ https://www.wiley.com/en-us/network/publishing/research-publishing/choosing-a-journal/6-steps-to-choosing-the-right-journal-for-your-research-infographic
- ↑ https://link.springer.com/article/10.1007/s13187-020-01751-z
- ↑ https://libguides.unomaha.edu/c.php?g=100510&p=651627
- ↑ https://www.canberra.edu.au/library/start-your-research/research_help/publishing-research
- ↑ https://writingcenter.fas.harvard.edu/conclusions
- ↑ https://writing.wisc.edu/handbook/assignments/writing-an-abstract-for-your-research-paper/
- ↑ https://www.springer.com/gp/authors-editors/book-authors-editors/your-publication-journey/manuscript-preparation
- ↑ https://apus.libanswers.com/writing/faq/2391
- ↑ https://academicguides.waldenu.edu/library/keyword/search-strategy
- ↑ https://ifis.libguides.com/journal-publishing-guide/submitting-your-paper
- ↑ https://www.springer.com/kr/authors-editors/authorandreviewertutorials/submitting-to-a-journal-and-peer-review/cover-letters/10285574
- ↑ https://www.apa.org/monitor/sep02/publish.aspx
- ↑ Matthew Snipp, PhD. Research Fellow, U.S. Bureau of the Census. Expert Interview. 26 March 2020.
About This Article

To publish a research paper, ask a colleague or professor to review your paper and give you feedback. Once you've revised your work, familiarize yourself with different academic journals so that you can choose the publication that best suits your paper. Make sure to look at the "Author's Guide" so you can format your paper according to the guidelines for that publication. Then, submit your paper and don't get discouraged if it is not accepted right away. You may need to revise your paper and try again. To learn about the different responses you might get from journals, see our reviewer's explanation below. Did this summary help you? Yes No
- Send fan mail to authors
Reader Success Stories

RAMDEV GOHIL
Oct 16, 2017
Did this article help you?

David Okandeji
Oct 23, 2019

Revati Joshi
Feb 13, 2017

Shahzad Khan
Jul 1, 2017

Apr 7, 2017

Featured Articles

Trending Articles

Watch Articles

- Terms of Use
- Privacy Policy
- Do Not Sell or Share My Info
- Not Selling Info
Don’t miss out! Sign up for
wikiHow’s newsletter
Home → Get Published → How to Publish a Research Paper: A Step-by-Step Guide
How to Publish a Research Paper: A Step-by-Step Guide
Jordan Kruszynski
- January 4, 2024
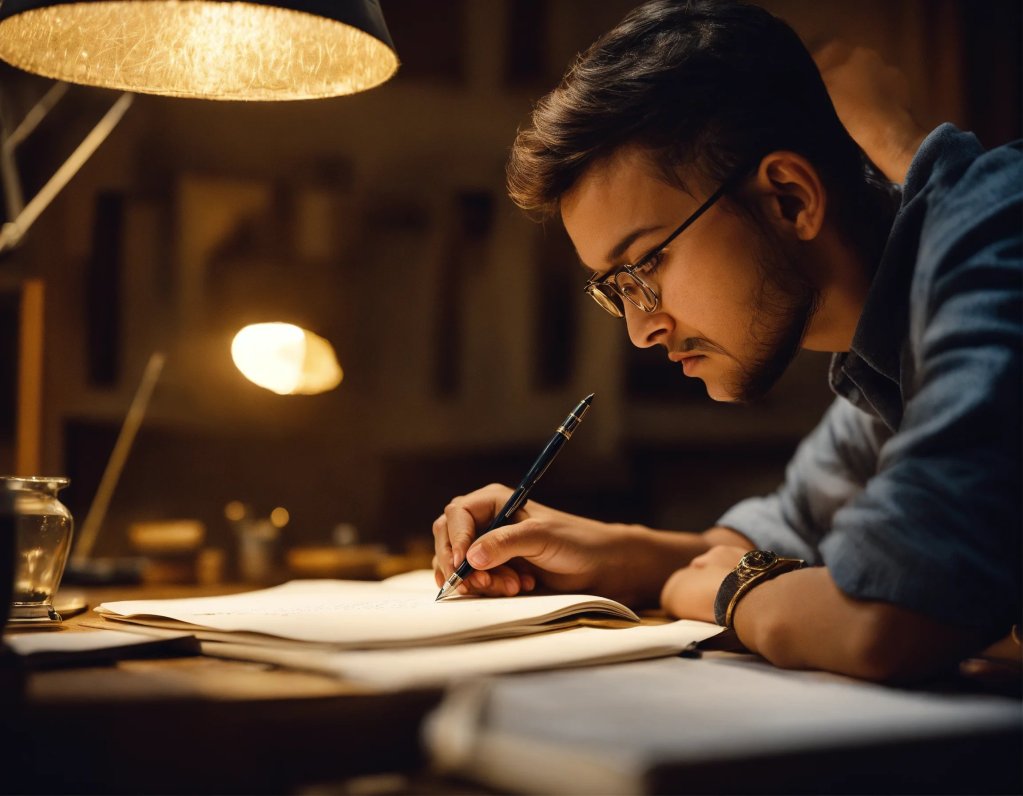
You’re in academia.
You’re going steady.
Your research is going well and you begin to wonder: ‘ How exactly do I get a research paper published?’
If this is the question on your lips, then this step-by-step guide is the one for you. We’ll be walking you through the whole process of how to publish a research paper.
Publishing a research paper is a significant milestone for researchers and academics, as it allows you to share your findings, contribute to your field of study, and start to gain serious recognition within the wider academic community. So, want to know how to publish a research paper? By following our guide, you’ll get a firm grasp of the steps involved in this process, giving you the best chance of successfully navigating the publishing process and getting your work out there.
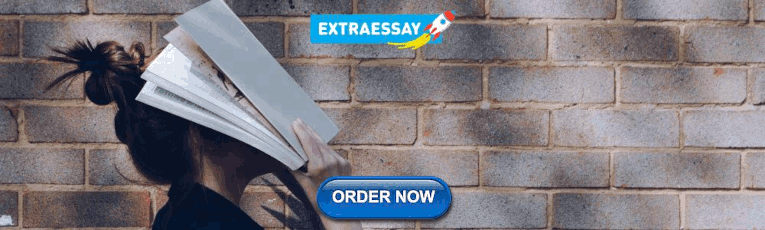
Understanding the Publishing Process
To begin, it’s crucial to understand that getting a research paper published is a multi-step process. From beginning to end, it could take as little as 2 months before you see your paper nestled in the pages of your chosen journal. On the other hand, it could take as long as a year .
Below, we set out the steps before going into more detail on each one. Getting a feel for these steps will help you to visualise what lies ahead, and prepare yourself for each of them in turn. It’s important to remember that you won’t actually have control over every step – in fact, some of them will be decided by people you’ll probably never meet. However, knowing which parts of the process are yours to decide will allow you to adjust your approach and attitude accordingly.
Each of the following stages will play a vital role in the eventual publication of your paper:
- Preparing Your Research Paper
- Finding the Right Journal
- Crafting a Strong Manuscript
- Navigating the Peer-Review Process
- Submitting Your Paper
- Dealing with Rejections and Revising Your Paper
Step 1: Preparing Your Research Paper
It all starts here. The quality and content of your research paper is of fundamental importance if you want to get it published. This step will be different for every researcher depending on the nature of your research, but if you haven’t yet settled on a topic, then consider the following advice:
- Choose an interesting and relevant topic that aligns with current trends in your field. If your research touches on the passions and concerns of your academic peers or wider society, it may be more likely to capture attention and get published successfully.
- Conduct a comprehensive literature review (link to lit. review article once it’s published) to identify the state of existing research and any knowledge gaps within it. Aiming to fill a clear gap in the knowledge of your field is a great way to increase the practicality of your research and improve its chances of getting published.
- Structure your paper in a clear and organised manner, including all the necessary sections such as title, abstract, introduction (link to the ‘how to write a research paper intro’ article once it’s published) , methodology, results, discussion, and conclusion.
- Adhere to the formatting guidelines provided by your target journal to ensure that your paper is accepted as viable for publishing. More on this in the next section…
Step 2: Finding the Right Journal
Understanding how to publish a research paper involves selecting the appropriate journal for your work. This step is critical for successful publication, and you should take several factors into account when deciding which journal to apply for:
- Conduct thorough research to identify journals that specialise in your field of study and have published similar research. Naturally, if you submit a piece of research in molecular genetics to a journal that specialises in geology, you won’t be likely to get very far.
- Consider factors such as the journal’s scope, impact factor, and target audience. Today there is a wide array of journals to choose from, including traditional and respected print journals, as well as numerous online, open-access endeavours. Some, like Nature , even straddle both worlds.
- Review the submission guidelines provided by the journal and ensure your paper meets all the formatting requirements and word limits. This step is key. Nature, for example, offers a highly informative series of pages that tells you everything you need to know in order to satisfy their formatting guidelines (plus more on the whole submission process).
- Note that these guidelines can differ dramatically from journal to journal, and details really do matter. You might submit an outstanding piece of research, but if it includes, for example, images in the wrong size or format, this could mean a lengthy delay to getting it published. If you get everything right first time, you’ll save yourself a lot of time and trouble, as well as strengthen your publishing chances in the first place.
Step 3: Crafting a Strong Manuscript
Crafting a strong manuscript is crucial to impress journal editors and reviewers. Look at your paper as a complete package, and ensure that all the sections tie together to deliver your findings with clarity and precision.
- Begin by creating a clear and concise title that accurately reflects the content of your paper.
- Compose an informative abstract that summarises the purpose, methodology, results, and significance of your study.
- Craft an engaging introduction (link to the research paper introduction article) that draws your reader in.
- Develop a well-structured methodology section, presenting your results effectively using tables and figures.
- Write a compelling discussion and conclusion that emphasise the significance of your findings.
Step 4: Navigating the Peer-Review Process
Once you submit your research paper to a journal, it undergoes a rigorous peer-review process to ensure its quality and validity. In peer-review, experts in your field assess your research and provide feedback and suggestions for improvement, ultimately determining whether your paper is eligible for publishing or not. You are likely to encounter several models of peer-review, based on which party – author, reviewer, or both – remains anonymous throughout the process.
When your paper undergoes the peer-review process, be prepared for constructive criticism and address the comments you receive from your reviewer thoughtfully, providing clear and concise responses to their concerns or suggestions. These could make all the difference when it comes to making your next submission.
The peer-review process can seem like a closed book at times. Check out our discussion of the issue with philosopher and academic Amna Whiston in The Research Beat podcast!
Step 5: Submitting Your Paper
As we’ve already pointed out, one of the key elements in how to publish a research paper is ensuring that you meticulously follow the journal’s submission guidelines. Strive to comply with all formatting requirements, including citation styles, font, margins, and reference structure.
Before the final submission, thoroughly proofread your paper for errors, including grammar, spelling, and any inconsistencies in your data or analysis. At this stage, consider seeking feedback from colleagues or mentors to further improve the quality of your paper.
Step 6: Dealing with Rejections and Revising Your Paper
Rejection is a common part of the publishing process, but it shouldn’t discourage you. Analyse reviewer comments objectively and focus on the constructive feedback provided. Make necessary revisions and improvements to your paper to address the concerns raised by reviewers. If needed, consider submitting your paper to a different journal that is a better fit for your research.
For more tips on how to publish your paper out there, check out this thread by Dr. Asad Naveed ( @dr_asadnaveed ) – and if you need a refresher on the basics of how to publish under the Open Access model, watch this 5-minute video from Audemic Academy !
Final Thoughts
Successfully understanding how to publish a research paper requires dedication, attention to detail, and a systematic approach. By following the advice in our guide, you can increase your chances of navigating the publishing process effectively and achieving your goal of publication.
Remember, the journey may involve revisions, peer feedback, and potential rejections, but each step is an opportunity for growth and improvement. Stay persistent, maintain a positive mindset, and continue to refine your research paper until it reaches the standards of your target journal. Your contribution to your wider discipline through published research will not only advance your career, but also add to the growing body of collective knowledge in your field. Embrace the challenges and rewards that come with the publication process, and may your research paper make a significant impact in your area of study!
Looking for inspiration for your next big paper? Head to Audemic , where you can organise and listen to all the best and latest research in your field!
Keep striving, researchers! ✨
Table of Contents
Related articles.
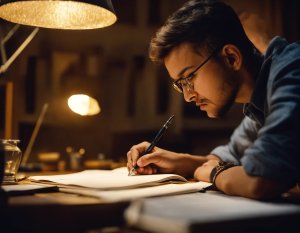
You’re in academia. You’re going steady. Your research is going well and you begin to wonder: ‘How exactly do I get a

Behind the Scenes: What Does a Research Assistant Do?
Have you ever wondered what goes on behind the scenes in a research lab? Does it involve acting out the whims of
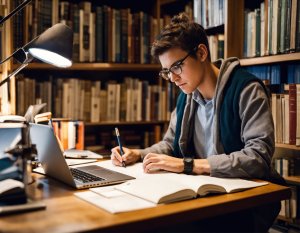
How to Write a Research Paper Introduction: Hook, Line, and Sinker
Want to know how to write a research paper introduction that dazzles? Struggling to hook your reader in with your opening sentences?

Blog Podcast
Privacy policy Terms of service
Subscribe to our newsletter!
Discover more from Audemic: Access any academic research via audio
Subscribe now to keep reading and get access to the full archive.
Type your email…
Continue reading
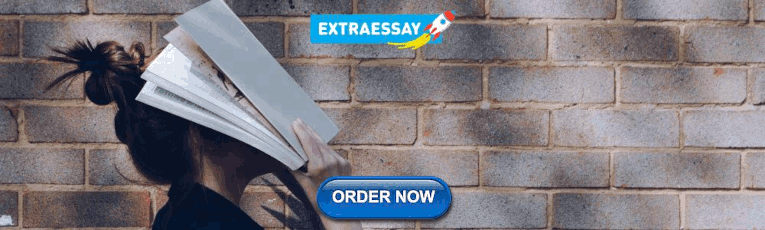
IMAGES
VIDEO