Academia.edu no longer supports Internet Explorer.
To browse Academia.edu and the wider internet faster and more securely, please take a few seconds to upgrade your browser .
Enter the email address you signed up with and we'll email you a reset link.
- We're Hiring!
- Help Center
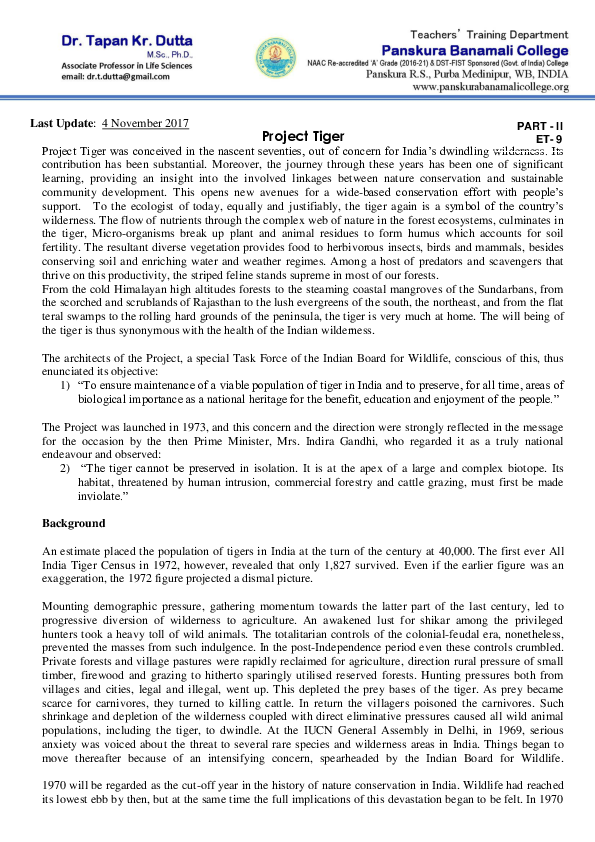
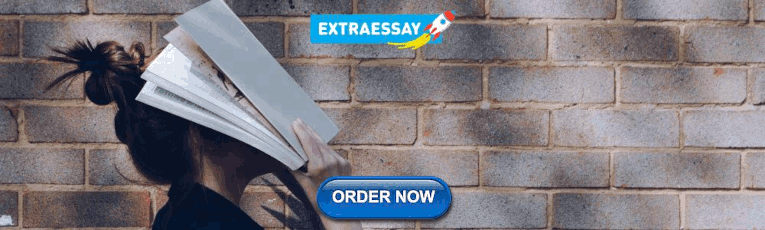
Project Tiger

Project Tiger was conceived in the nascent seventies, out of concern for India's dwindling wilderness. Its contribution has been substantial. Moreover, the journey through these years has been one of significant learning, providing an insight into the involved linkages between nature conservation and sustainable community development. This opens new avenues for a wide-based conservation effort with people's support. To the ecologist of today, equally and justifiably, the tiger again is a symbol of the country's wilderness. The flow of nutrients through the complex web of nature in the forest ecosystems, culminates in the tiger, Microorganisms break up plant and animal residues to form humus which accounts for soil fertility. The resultant diverse vegetation provides food to herbivorous insects, birds and mammals, besides conserving soil and enriching water and weather regimes. Among a host of predators and scavengers that thrive on this productivity, the striped feline stands supreme in most of our forests. From the cold Himalayan high altitudes forests to the steaming coastal mangroves of the Sundarbans, from the scorched and scrublands of Rajasthan to the lush evergreens of the south, the northeast, and from the flat teral swamps to the rolling hard grounds of the peninsula, the tiger is very much at home. The will being of the tiger is thus synonymous with the health of the Indian wilderness. The architects of the Project, a special Task Force of the Indian Board for Wildlife, conscious of this, thus enunciated its objective: 1) " To ensure maintenance of a viable population of tiger in India and to preserve, for all time, areas of biological importance as a national heritage for the benefit, education and enjoyment of the people. " The Project was launched in 1973, and this concern and the direction were strongly reflected in the message for the occasion by the then Prime Minister, Mrs. Indira Gandhi, who regarded it as a truly national endeavour and observed: 2) " The tiger cannot be preserved in isolation. It is at the apex of a large and complex biotope. Its habitat, threatened by human intrusion, commercial forestry and cattle grazing, must first be made inviolate. " Background
Related Papers
Surjit Bhujabal
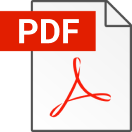
Global Ecology and Conservation
Vasanth Reddy
Mohammed Ashraf
The tiger is a breathtakingly charismatic species. It is the largest terrestrial carnivorous mammal on earth and sits on top of the food chain in tropical, grassland and boreal ecosystem s ranging from South Asia to Central Asia and Siberia in the former USSR. In these eco-regions, the tiger has served as a flagship mammal and hence acted as an umbrella species for overall biodiversity conservation management. It is an ecological indicator species from which wildlife biologists can detect various ecological changes in terms of species richness, size, equitability, biomass, etc. Some wildlife biologists refer to the tiger as an ecological litmus paper because of its role in helping to indicate the overall health of tropical ecosystem s in South and South-East Asia.
Odisha Wildlife Conclave 2023
Lala Aswini Kumar Singh
The talk is at: https://youtu.be/OgOoDZ8eW0M It is about tiger conservation in Odisha state, which contributed tiger enumeration technique to India in 1972; it is one of the first nine states which launched Project Tiger in 1973; and have warned about population stagnation with imminent population decline from 1995-96; and cautioned about the appearance of melanistic tiger in Similipal. The warning and cautions proved right after 27-28 years. The talk gave advice to officers in charge of wildlife and tiger conservation.
World Bank Publications
Biodiversity is critical to maintaining the integrity of ecosystems and the ecological processes that support species and human well-being. The world is facing an unprecedented rate of species extinction: one in eight bird species, one in four mammals, and one in three amphibians are threatened. Species can recover with concerted conservation. As a charismatic endangered species, tigers have become a powerful symbol of biodiversity loss globally, as their numbers have dropped from 100,000 at the turn of the 20th century to an estimated 3,000-3,500 tigers in the wild today. The need to protect tigers has taken on great urgency, and international efforts are attempting to pull them back from the edge of extinction. From November 21 to 24, 2010, the Russian Federation hosted leaders from 13 tiger range countries at a conference in St. Petersburg. The goal was inter alia to eliminate illegal trade in tiger parts while protecting tiger habitats and to double the tiger population by 2022....
Alexandra Vladimirova
Bikku Rathod
Conservation Genetics
Jyotsna Bhagavatula , Katakam Harika , sisinthy shivaji
Frontiers in Ecology and Evolution
Gautam Talukdar
Loading Preview
Sorry, preview is currently unavailable. You can download the paper by clicking the button above.
RELATED PAPERS
Dipendra Adhikari , Babu R Lamichhane , Naresh Subedi
Prasanta Kumar Saikia
Priya Singh
Jimmy Borah
Qamar Qureshi
Nazeem Quazi
Gosia (Malgorzata) Bryja
Ashwin Naidu , Kenneth Walker
Jayanta Mallick
Frontiers in conservation science
Channa Phan
Samrat Mondol
Maheshwar Dhakal
Vasanth Kamath
Journal of Threatened Taxa
Abishek Harihar
Environmental Conservation
Nigel Leader-williams
C R E W M B R
udit sharma
Sudha Vasan
Shekhar Kolipaka
The Initiation
Jhamak B Karki
RELATED TOPICS
- We're Hiring!
- Help Center
- Find new research papers in:
- Health Sciences
- Earth Sciences
- Cognitive Science
- Mathematics
- Computer Science
- Academia ©2024
Tiger Conservation in India - Project Tiger
Khandelwal, V. 2005. Tiger Conservation in India. New Delhi: Centre for Civil Society
62 Pages Posted: 9 Aug 2005
Varun Khandelwal
Centre for Civil Society
The primary focus of the paper is to analyze the Indian Tiger Conservation program - Project Tiger. The circumstances that lead to and the conditions under which the program was launched are discussed. National Parks in India lead to a situation where the natives of the forest are deprived of their homes and livelihoods as an externality of conservation. These ousted populations ('green oustees') often turn against the National Parks for their sustenance. The first fifteen years of the program showed spectacular rises in tiger populations. Demographic trends of tiger populations in India had peaked around 1987. The method of census - the pugmark method, is far from reliable. Project Tiger suffers from various problems - delayed and inadequate funds, poaching, grazing, encroachment and inadequacy of staff both in training and in numbers. Project Tiger Status Report 2001 acknowledges these issues. There are instances when Project Tiger National Parks receive funds at the end of the financial year due to the long bureaucratic process involved in sanctioning and transfer of funds. This results in the ability of Project Tiger National Parks to utilize all the funds that are allocated to them. The National Parks have inadequately trained and insufficient number of field staff. Special emphasis has been laid on the problem of the delayed delivery of funds. The 'money trail' has been analyzed - no less than seven different Government departments are involved in the process that delivers the funds to the National Parks. These, too, may be involved more than once. The delayed receipt of funds is vitally detrimental to the performance of the parks. The paper gives recommendations for improving the process of sanction and delivery of funds to Project Tiger National Parks. Various players in the Indian case are enumerated. The goal of saving the tiger can, in the long-term, be accomplished better by a shift of the Government's role from keeper to regulator.
Keywords: Tiger, conservation, project tiger, national parks, centrally sponsored schemes, demographic trends, flow of funds, staff, annual plan of operation, wildlife protection act, 1972
JEL Classification: Q38, Q28, K32
Suggested Citation: Suggested Citation
Varun Khandelwal (Contact Author)
Centre for civil society ( email ).
K-36 Hauz Khas Enclave New Delhi 110 016, KwaZulu-Natal India
Do you have a job opening that you would like to promote on SSRN?
Paper statistics, related ejournals, built environment ejournal.
Subscribe to this fee journal for more curated articles on this topic
Sustainability & Economics eJournal
Environmental economics ejournal, india law ejournal, environmental law & policy ejournal, natural resources law & policy ejournal, protected lands law & policy ejournal.
- Department Login

- Research Papers
Camera trap placement for evaluating species richness, abundance, and activity
Information from camera traps is used for inferences on species presence, richness, abundance, demography, and activity. Camera trap placement design
Demography of a small, isolated tiger (Panthera tigris tigris) population in a semi- arid region of western India
Background: Tiger populations have declined globally due to poaching, prey depletion, and habitat loss. The westernmost tiger population of Ranthambhore
Distribution, Status, and Conservation of the Indian Peninsular Wolf
An understanding of the distribution range and status of a species is paramount for its conservation. We used photo captures
Do conservation strategies that increase tiger populations have consequences for other wild carnivores like leopards?
Most large carnivore populations are declining across their global range except in some well managed protected areas (PA’s). Investments for
Genetic structure of tigers (Panthera tigris tigris) in India and its implications for conservation
Identifying and prioritising naturally occurring within-species diversity, which may correlate with local adaptations or vicariance, is an integral part of
Demography of a high‐density tiger population and its implications for tiger recovery
Prioritizing conservation of source populations within landscapes is proposed as a strategy for recovering tigers globally. We studied population dynamics
Schrodinger’s scat: a critical review of the currently available tiger (Panthera Tigris) and leopard (Panthera pardus) specific primers in India, and a novel leopard
Background: Non-invasive sampling has opened avenues for the genetic study of elusive species, which has contributed significantly to their conservation.
Twisted tale of the tiger: the case of inappropriate data and deficient science
Publications in peer-reviewed journals are often looked upon as tenets on which future scientific thought is built. Published information is
Density and Distribution of Principal Prey Species of Tigers and Leopards in Pench Tiger Reserve, Madhya Pradesh
Conservation of large carnivores is dependent on a viable population of their principal prey species. Line transect based density estimation
Other Publications
Habitat plots and sampling, polygon search method, dos and don’ts for sign survey and transect walks, camera trapping protocol, line transect sampling for prey estimation, carnivore sign survey: protocols and data collection.

Quick Links
- Annoucements
- Tiger Reports
Prof. Qamar Qureshi, Wildlife Institute Of India (WII), Chandrabani, Dehradun - 248001, Uttarakhand
- mstripes[dot]team[at]wii[dot]gov[dot]in
- +91 135 2646181
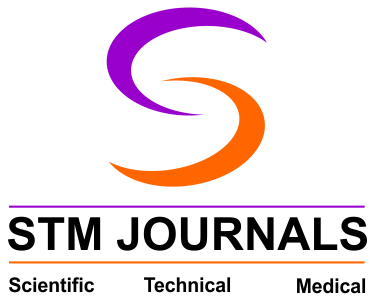
- STM Journals
- Special Issues
- Conferences
- Editorial Board Members
- Reviewers Board Members
- Advisory Panel
- Indexing Bodies
- For Authors
- For Reviewers
- For Editors
- For Advisory Board
- Special Issue Guidelines
- Peer-Review Policy
- Manuscript Submission and Processing
- Publication Ethics and Virtue
- Article Processing Charge
- Editorial Policy
- Advertising Policy
- STM Website and Link Policy
- Distribution and dessemination of Research
- Informed consent Policy
- DOI Payment
"Connect with colleagues and showcase your academic achievements."
"Unleashing the potential of your words"
"Explore a vast collection of books and broaden your horizons."
"Empower yourself with the knowledge and skills needed to succeed."
"Collaborate with like-minded professionals and share your knowledge."
"Learn from experts and engage with a community of learners."
- ICDR Group of Companies
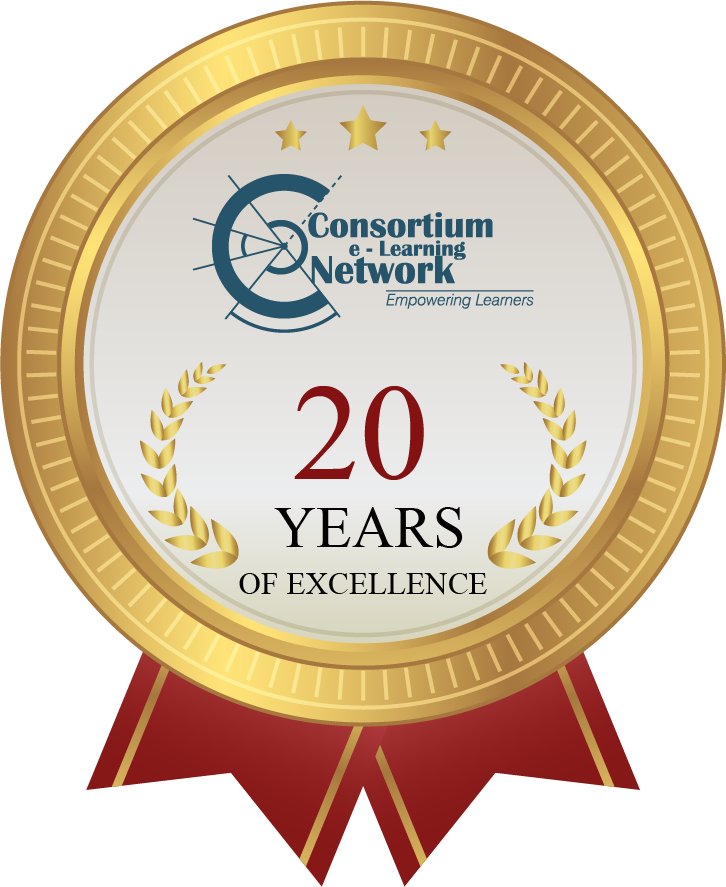
- Training Programs
A Study on Project Tiger in India
Open Access
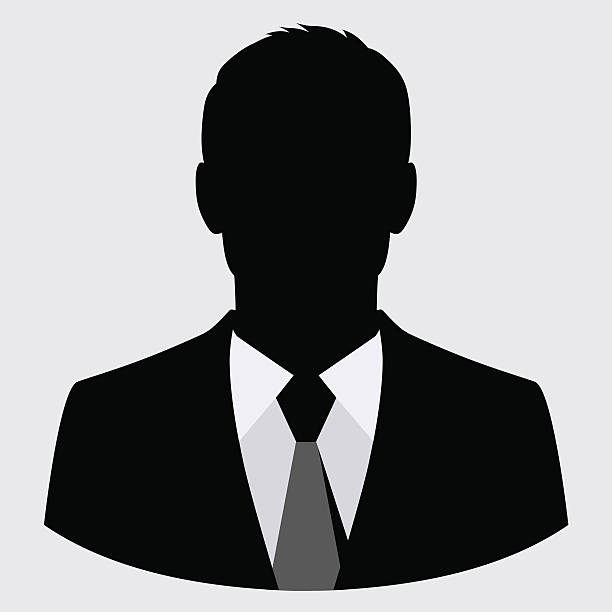
B.S Yashaswini,
- Student Christ (Deemed to be University), Bangalore Karnataka India
This research paper analyses the illegal wildlife trade in India, where many animals and related items are dispatched daily as food, pets, medications, clothing, and trophies which threatens plants and animals to the verge of extinction and obstructs sustainable development leading to corruption and criminality. It mentions The Project Tiger, launched by the Government of India in 1973 to ensure Bengal tigers’ population and maintain their natural habitats, including setting up a tiger protecting force. The paper further examines the effectiveness of India’s forest and wildlife laws. It analyzes the efficiency of the forest and wildlife legislations including the analysis of the various challenges faced while protecting wildlife and forestry and the Government’s strategies while framing wildlife policies.
Keywords: Tiger conservation, Project Tiger in India, Illegal Wildlife Trade, Wildlife Policies, Bengal Tigers
Full Text PDF Download
Fetching IP address…
1. Report- Jhala, Y. V., Gopal, R., Qureshi, Q. (eds.) (2008). Status of the Tigers, Co-predators, and Prey in India (PDF). TR 08/001. National Tiger Conservation Authority, Govt. of India, New Delhi; Wildlife Institute of India, Dehradun. Archived from the original (PDF) on 2 June 2013.(Last visited on October-13-2021) 2. Fresh air report-Ambassador report( Last visited on october-12-2021) ( Last accessed on 14th October, 2021 12.30 P.M) https://tunza.ecogeneration.org/ambassadorReportView.jsp?viewID=4375 3. Block-Indian Wildlife Tour with Taj Mahal Tiger Safari through the wilderness of India (Last visited on October-12-2021) (2.00 p.m) Project Tiger in India | Tiger Reserves India 4. International Journal of Science, Engineering and Technology-Ashish Sharma et al. 2018, Volume 6 Issue 1 ISSN (Online): A Geographical Study of Tiger Projects in India ( Last visited on October-13-2021) https://www.ijset.in/wpcontent/uploads/10.2348.ijset01180122.pdf 5. Justice P.N. Bhagwati Lectures on India’s human rights law, Columbia Law Schoohttps://www.law.columbia.edu/news/archive/justice-pn-bhagwati-lectures-indias-humanrights-law (last visited Oct 15, 2021)
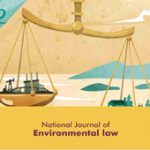
National Journal of Environmental law
January 11, 2022 | |
January 15, 2022 | |
January 15, 2023 |
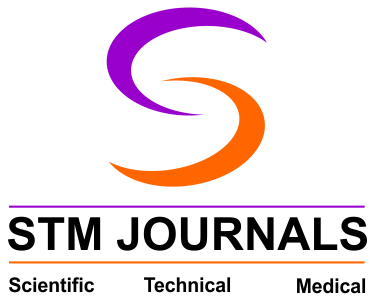
WEBSITE DISCLAIMER
Last updated: 2022-06-15
The information provided by STM Journals (“Company”, “we”, “our”, “us”) on https://journals.stmjournals.com / (the “Site”) is for general informational purposes only. All information on the Site is provided in good faith, however, we make no representation or warranty of any kind, express or implied, regarding the accuracy, adequacy, validity, reliability, availability, or completeness of any information on the Site.
UNDER NO CIRCUMSTANCE SHALL WE HAVE ANY LIABILITY TO YOU FOR ANY LOSS OR DAMAGE OF ANY KIND INCURRED AS A RESULT OF THE USE OF THE SITE OR RELIANCE ON ANY INFORMATION PROVIDED ON THE SITE. YOUR USE OF THE SITE AND YOUR RELIANCE ON ANY INFORMATION ON THE SITE IS SOLELY AT YOUR OWN RISK.
EXTERNAL LINKS DISCLAIMER
The Site may contain (or you may be sent through the Site) links to other websites or content belonging to or originating from third parties or links to websites and features. Such external links are not investigated, monitored, or checked for accuracy, adequacy, validity, reliability, availability, or completeness by us.
WE DO NOT WARRANT, ENDORSE, GUARANTEE, OR ASSUME RESPONSIBILITY FOR THE ACCURACY OR RELIABILITY OF ANY INFORMATION OFFERED BY THIRD-PARTY WEBSITES LINKED THROUGH THE SITE OR ANY WEBSITE OR FEATURE LINKED IN ANY BANNER OR OTHER ADVERTISING. WE WILL NOT BE A PARTY TO OR IN ANY WAY BE RESPONSIBLE FOR MONITORING ANY TRANSACTION BETWEEN YOU AND THIRD-PARTY PROVIDERS OF PRODUCTS OR SERVICES.
PROFESSIONAL DISCLAIMER
The Site can not and does not contain medical advice. The information is provided for general informational and educational purposes only and is not a substitute for professional medical advice. Accordingly, before taking any actions based on such information, we encourage you to consult with the appropriate professionals. We do not provide any kind of medical advice.
Content published on https://journals.stmjournals.com / is intended to be used and must be used for informational purposes only. It is very important to do your analysis before making any decision based on your circumstances. You should take independent medical advice from a professional or independently research and verify any information that you find on our Website and wish to rely upon.
THE USE OR RELIANCE OF ANY INFORMATION CONTAINED ON THIS SITE IS SOLELY AT YOUR OWN RISK.
AFFILIATES DISCLAIMER
The Site may contain links to affiliate websites, and we may receive an affiliate commission for any purchases or actions made by you on the affiliate websites using such links.
TESTIMONIALS DISCLAIMER
The Site may contain testimonials by users of our products and/or services. These testimonials reflect the real-life experiences and opinions of such users. However, the experiences are personal to those particular users, and may not necessarily be representative of all users of our products and/or services. We do not claim, and you should not assume that all users will have the same experiences.
YOUR RESULTS MAY VARY.
The testimonials on the Site are submitted in various forms such as text, audio, and/or video, and are reviewed by us before being posted. They appear on the Site verbatim as given by the users, except for the correction of grammar or typing errors. Some testimonials may have been shortened for the sake of brevity, where the full testimonial contained extraneous information not relevant to the general public.
The views and opinions contained in the testimonials belong solely to the individual user and do not reflect our views and opinions.
ERRORS AND OMISSIONS DISCLAIMER
While we have made every attempt to ensure that the information contained in this site has been obtained from reliable sources, STM Journals is not responsible for any errors or omissions or the results obtained from the use of this information. All information on this site is provided “as is”, with no guarantee of completeness, accuracy, timeliness, or of the results obtained from the use of this information, and without warranty of any kind, express or implied, including, but not limited to warranties of performance, merchantability, and fitness for a particular purpose.
In no event will STM Journals, its related partnerships or corporations, or the partners, agents, or employees thereof be liable to you or anyone else for any decision made or action taken in reliance on the information in this Site or for any consequential, special or similar damages, even if advised of the possibility of such damages.
GUEST CONTRIBUTORS DISCLAIMER
This Site may include content from guest contributors and any views or opinions expressed in such posts are personal and do not represent those of STM Journals or any of its staff or affiliates unless explicitly stated.
LOGOS AND TRADEMARKS DISCLAIMER
All logos and trademarks of third parties referenced on https://journals.stmjournals.com / are the trademarks and logos of their respective owners. Any inclusion of such trademarks or logos does not imply or constitute any approval, endorsement, or sponsorship of STM Journals by such owners.
Should you have any feedback, comments, requests for technical support, or other inquiries, please contact us by email: [email protected] .
Thank you for visiting nature.com. You are using a browser version with limited support for CSS. To obtain the best experience, we recommend you use a more up to date browser (or turn off compatibility mode in Internet Explorer). In the meantime, to ensure continued support, we are displaying the site without styles and JavaScript.
- View all journals
- Explore content
- About the journal
- Publish with us
- Sign up for alerts
- NEWS FEATURE
- 30 October 2019
India’s tigers seem to be a massive success story — many scientists aren’t sure
- Gayathri Vaidyanathan 0
Gayathri Vaidyanathan is a science journalist in Bengaluru.
You can also search for this author in PubMed Google Scholar
A tiger and her cubs leave India’s Bandhavgarh National Park in search of prey, which is scarce inside the reserve. Credit: Steve Winter/National Geographic
Central India — The Maruti Gypsy 44 sped along a jungle track, jolting us out of our seats. We had signed up for a wolf safari, but the trip leader had another quarry in mind. The vehicle barrelled towards a pungent smell on a hillside — a fresh tiger kill.
Access options
Access Nature and 54 other Nature Portfolio journals
Get Nature+, our best-value online-access subscription
24,99 € / 30 days
cancel any time
Subscribe to this journal
Receive 51 print issues and online access
185,98 € per year
only 3,65 € per issue
Rent or buy this article
Prices vary by article type
Prices may be subject to local taxes which are calculated during checkout
Nature 574 , 612-616 (2019)
doi: https://doi.org/10.1038/d41586-019-03267-z
Mondol, S., Karanth, K. U. & Ramakrishnan, U. PLoS Genet. 5 , e1000585 (2009).
Article PubMed Google Scholar
Gopalaswamy, A. M., Delampady, D., Karanth, K. U., Kumar, N. S. & Macdonald, D. W. Methods Ecol. Evol. 6 , 1055–1066 (2015).
Article Google Scholar
Gopalaswamy, A. M., Karanth, K. U., Delampady, M. & Stenseth, N. Chr. Preprint at bioRxiv https://doi.org/10.1101/708628 (2019).
Qureshi, Q., Gopal, R. & Jhala, Y. PeerJ 7 , e7482 (2019).
Thatte, P., Joshi, A., Vaidyanathan, S., Landguth, E. & Ramakrishnan, U. Biol. Conserv. 218 , 181–191 (2018).
Armstrong, E. et al. Preprint at bioRxiv https://doi.org/10.1101/696146 (2019).
Kerley, L. L. et al. Conserv. Biol. 16 , 97–108 (2002).
Download references
Reprints and permissions
Related Articles
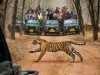
- Conservation biology
- Biodiversity
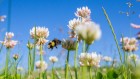
Bumblebees’ sense of smell can’t take the heat
Research Highlight 30 AUG 24
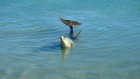
Lonely? Playful? Why are dolphin attacks rising in Japan?
News Q&A 28 AUG 24
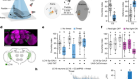
Mating proximity blinds threat perception
Article 28 AUG 24
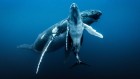
Dismantle ‘zombie’ wildlife protection conventions once their work is done
Comment 12 AUG 24
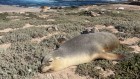
Camera-carrying sea lions map uncharted areas of the ocean
News 07 AUG 24
Tenure-Track/Tenured Faculty Positions
Tenure-Track/Tenured Faculty Positions in the fields of energy and resources.
Suzhou, Jiangsu, China
School of Sustainable Energy and Resources at Nanjing University
ATLAS - Joint PhD Program from BioNTech and TRON with a focus on translational medicine
5 PhD positions for ATLAS, the joint PhD Program from BioNTech and TRON with a focus on translational medicine.
Mainz, Rheinland-Pfalz (DE)
Translational Oncology (TRON) Mainz
Alzheimer's Disease (AD) Researcher/Associate Researcher
Xiaoliang Sunney XIE’s Group is recruiting researchers specializing in Alzheimer's disease (AD).
Beijing, China
Changping Laboratory
Supervisory Bioinformatics Specialist CTG Program Head
The National Library of Medicine (NLM) is a global leader in biomedical informatics and computational health data science and the world’s largest b...
Bethesda, Maryland (US)
National Library of Medicine, National Center for Biotechnology Information
Post Doctoral Research Scientist
Post-Doctoral Research Scientist Position in Human Transplant Immunology at the Columbia Center for Translational Immunology in New York, NY
New York City, New York (US)
Columbia Center for Translational Immunoogy
Sign up for the Nature Briefing newsletter — what matters in science, free to your inbox daily.
Quick links
- Explore articles by subject
- Guide to authors
- Editorial policies

An official website of the United States government
The .gov means it’s official. Federal government websites often end in .gov or .mil. Before sharing sensitive information, make sure you’re on a federal government site.
The site is secure. The https:// ensures that you are connecting to the official website and that any information you provide is encrypted and transmitted securely.
- Publications
- Account settings
Preview improvements coming to the PMC website in October 2024. Learn More or Try it out now .
- Advanced Search
- Journal List
- Animals (Basel)

New Evidence of Tiger Subspecies Differentiation and Environmental Adaptation: Comparison of the Whole Genomes of the Amur Tiger and the South China Tiger
1 College of Wildlife and Protected Area, Northeast Forestry University, Harbin 150040, China; moc.361@2109rhd (H.D.); moc.361@7891uynijnij (J.Y.)
Jingjing Yu
2 Resources & Environment College, Tibet Agricultural and Animal Husbandry University, Nyingchi 860000, China
3 College of Pharmacy, Guizhou University of Traditional Chinese Medicine, Guiyang 550025, China
Minghai Zhang
Associated data.
Raw sequence data were submitted to the NCBI Sequence Read Archive (SRA) under accession number SRP156517 (SRX4514236, SRX4514235, SRX4514234, SRX4514233, SRX4514232, SRX4514231, SRX4514230, SRX4514229).
Simple Summary
Tigers are top predators and umbrella protectors, vital to the stability of ecosystems. The South China tiger has been declared extinct in the wild and only exists in captivity. The Chinese government is actively promoting the reintroduction of the South China tiger into the wild. The future of the wild population of the Amur tiger in China is not optimistic, and the recovery of the population is an essential task for the conservation of the Amur tiger. The recovery of the population is not only a macroscopic problem but also a significant study of molecular ecology. We used high-throughput sequencing technology to study the differences in adaptive selection between Amur tigers and South China tigers. Significant genetic differences were found between the Amur tiger and the South China tiger based on a principal component analysis and phylogenetic tree. We identified functional genes and regulatory pathways related to reproduction, disease, predation, and metabolism and characterized functional genes related to survival in the wild, such as smell, vision, muscle, and predatory ability. The data also provide new evidence for the adaptation of Amur tigers to cold environments. PRKG1 is involved in temperature regulation in a cold climate. FOXO1 and TPM4 regulate body temperature to keep it constant. The research also provides a molecular basis for future tiger conservation.
Panthera tigris is a top predator that maintains the integrity of forest ecosystems and is an integral part of biodiversity. No more than 400 Amur tigers ( P. t. altaica ) are left in the wild, whereas the South China tiger ( P. t. amoyensis ) is thought to be extinct in the wild, and molecular biology has been widely used in conservation and management. In this study, the genetic information of Amur tigers and South China tigers was studied by whole-genome sequencing (WGS). A total of 647 Gb of high-quality clean data was obtained. There were 6.3 million high-quality single-nucleotide polymorphisms (SNPs), among which most (66.3%) were located in intergenic regions, with an average of 31.72% located in coding sequences. There were 1.73 million insertion-deletions (InDels), among which there were 2438 InDels (0.10%) in the coding region, and 270 thousand copy number variations (CNVs). Significant genetic differences were found between the Amur tiger and the South China tiger based on a principal component analysis and phylogenetic tree. The linkage disequilibrium analysis showed that the linkage disequilibrium attenuation distance of the South China tiger and the Amur tiger was almost the same, whereas the r 2 of the South China tiger was 0.6, and the r 2 of the Amur tiger was 0.4. We identified functional genes and regulatory pathways related to reproduction, disease, predation, and metabolism and characterized functional genes related to survival in the wild, such as smell, vision, muscle, and predatory ability. The data also provide new evidence for the adaptation of Amur tigers to cold environments. PRKG1 is involved in temperature regulation in a cold climate. FOXO1 and TPM4 regulate body temperature to keep it constant. Our results can provide genetic support for precise interspecies conservation and management planning in the future.
1. Introduction
Rapid human population growth, environmental change, and habitat fragmentation pose ever-greater biodiversity threats and highlight the need for increasingly aggressive conservation efforts (e.g., focused on Panthera tigris ). The tiger is the largest cat [ 1 ] and one of the most endangered species in the world. Currently, the world’s wild individual tigers number from about 2154 to 3159, and most of them are isolated in small populations [ 2 ]. The tiger is the key species and natural indicator of the health of ecological communities [ 3 ]. Historically, the tiger consisted of nine genetically validated subspecies [ 4 , 5 ], but only five subspecies (Amur, Bengal, Indochinese, Malayan, and Sumatran tigers) remain now [ 5 ]. In April of 2007, the Chinese government announced that there were no traces of South China tiger ( P. t. amoyensis ) activity in the wild [ 6 ]. Although144 individuals are in captivity [ 7 ], their ancestors are six individuals captured from the field. Inbreeding, low genetic diversity, minimal populations, and endangerment currently block South China tiger populations from recovery. Appropriate artificial interference may preserve the population, although low genetic diversity makes it difficult to restore the minimal populations and release them into the wild [ 8 ].
Molecular biology technology has been widely used in biological protection, especially the protection of endangered animals and plants [ 9 , 10 , 11 , 12 ]. Whole-genome sequencing (WGS) provides insights into genetic information recorded in gene fragments, such as historical population dynamics of species, evolutionary bottlenecks, hybridization, gene penetration, genetic diversity, inbreeding in small populations, etc. Population recovery is an effective way to save endangered species. Currently, the South China tiger is considered extinct in the wild. Only 204 individuals (by the end of 2019) exist in ex situ conservation, all of which were bred from the initial six individuals. [ 13 ]. Although the population of captive South China tigers has increased [ 13 , 14 ], inbreeding is inevitable due to the small initial population [ 8 , 15 ]. The dangers of inbreeding are apparent, especially for the South China tiger, which is extinct in the wild [ 16 ]. Meanwhile, the South China tiger’s importance as a top predator in the ecosystem is so great that the Chinese government is actively promoting its reintroduction into the wild [ 17 ]. Genomic tools facilitate the management of captive populations and the selection of individuals to be released by providing accurate genetic parameters [ 18 ].
The future of the wild population of the Amur tiger ( P. t. altaica ) is not optimistic, and population recovery is also an important issue currently facing these tigers. The genome study offers practical molecular biological support for the population of Amur tigers. We consider the most successful case of endangered species recovery to be the genetic recovery of the endangered Florida panther ( Puma concolor coryi ) population [ 19 ]. We hope that the recovery of the South China tiger population can be guided by gene rescue, which is based on the screening of the genome. Therefore, a genome-wide study is significant for the conservation management and population recovery of Amur and South China tigers.
The genomes of the Amur tiger and the South China tiger were studied by using whole-genome sequencing technology. We explored: (1) How did the chronological sequence of the Amur tigers and South China tigers evolve? (2) Which functional genes are associated with tiger survival in the wild and can be found by studying functional genes and regulatory channels? The results can provide a scientific reference for the molecular conservation and management of captive Amur tigers and South China tigers in the future and lay the foundation of genetic information for population recovery by providing insights for releasing the selection of individual tigers into the wild.
2. Materials and Methods
2.1. sample collection.
In this study, a total of seven tissue samples and one blood sample were collected for whole-genome sequencing. The Amur tiger samples were donated from the Northeast Tiger Park in northeastern Heilongjiang province and the South China tigers were donated from Shanghai Zoo. The experimental samples were placed in the refrigerator at −20 °C after collection. The detailed information of the eight samples is shown in Supplementary Table S1 .
2.2. DNA Extraction and Construction of a Genome Library
Tissue and blood samples were collected, and the DNA of each sample was extracted from tissue samples using standard phenol–chloroform methods. The steps were as follows: (1) ground the tissue samples to powder; (2) dissolve the tissue powder with STE, then add protease K to obtain the final concentration of 100 μg/mL; (3) shake in a 55 °C water bath overnight for digestion; (4) add the same volume of phenol and extract 1 time, then follow by shaking for 5 min and centrifugation at 12,000× g for 5 min; (5) take the supernatant and add phenol:chloroform:isopentyl alcohol (25:24:1), extract once, shake slightly for 5 min, and centrifuge 12,000× g for 5 min; (6) take the supernatant and add chloroform:isoamyl alcohol (24:1) to extract once and centrifuge; (7) take the supernatant, add iced ethanol two times and 1/10 of 3M sodium acetate, thoroughly mix 12,000× g by centrifugation for 5 min, then remove the supernatant; (8) rinse twice with 500μg of 75% ethanol; (9) finally, dissolve dd H20. Blood samples were extracted using a QIAamp DNA Mini Kit (Qiagen, https://www.qiagen.com/cn/shop/automated-solutions/qiaamp-dna-blood-mini-kit ) (accessed on 1 August 2020).
The qualified DNA samples were randomly interrupted by a Covaris ultrasonic crusher, the fragment ends were repaired, and a poly(A) tail was added. Sequencing joints were connected, purification, PCR amplification, and filtering were completed, and the whole library was prepared. After the library was qualified, Illumina HiSeq/MiSeq sequencing was performed by pooling different libraries according to the requirements of effective concentration and the target data amount. The constructed library was double-ended by an Illumina Hiseq PE150 sequencer, and the whole genome of reads with a length of 150 bp was sequenced. The sequencing depth was targeted at an average of >25×, and the average coverage was 99. 82% (coverage of at least one base), producing an average of 77.01 Gb of raw sequencing data per individual.
2.3. Resequencing and Variant Identification
Total genomic DNA from 8 tigers was extracted as described by Diversity Arrays Technology for Illumina sequencing. Paired-end sequencing libraries with insert sizes of 150 bp were constructed according to the manufacturer’s instructions for sequencing on the HiSeq PE150 platform. Paired-end reads (clean reads) obtained from sequencing were mapped to the Amur tiger genome [ 20 ] using the MEM algorithm from the BWA software [ 21 ]. The parameters used were “mem -t 4 -k 32 -M”.
SAMtools [ 22 ] was used to convert mapping results to bam format. The bam alignments were then converted to pileup and glf formats using the pileup command. SNPs and InDels were then detected using BCFtools; the Bayesian model was used to determine the base’s mutation type and polymorphism loci. Sites with a probability to be a variant >0.99 were further extracted to identify the putative SNP based on the following criteria: copy number <1.5, sequencing depth according to an average depth of each accession, 1000 < sequencing depth <3000, and SNPs a minimum of 5 bp apart, except for minor allele frequencies (MAF >0.05). ANNOVAR [ 23 ] was used to calculate the likelihood of genotypes of each individual. In each individual, SNPs were filtered by the quality value (>20), the minimum number of required reads supporting each SNP (>4), the maximum overall depth (<100), the maximum copy number of flanking sequences (<1.5), and the p -value of the rank sum test ( p > 0.05).
2.4. Copy Number Variation
Copy number variations (CNVs), which include deletions, duplications, and large-scale copy number variants or copy-number polymorphisms as well as insertions, inversions, and translocations, are believed to contribute significantly to variations between individuals and may have as large an effect on phenotype as SNPs [ 24 ]. CNVnator [ 25 ] (parameters “-call 100”) was used to detect and screen to obtain the number, structure, and location of CNVs. Read depth per 100 bp window was computed using this modified software, which adjusts for bias in reading depth caused by GC content, and mutation annotation was performed through the ANNOVAR (ANNOtate VARiation) tool [ 23 , 26 ].
2.5. Calculation of LD
Correlation coefficient values (r 2 ) of alleles were calculated using Haploview to measure the LD level in the two populations. The parameters were set as follows: “-dprime -max Distance 1000 -min MAF 0.05 -hwcutoff 0.001 -missing Cutoff 0.5 -min Geno 0.6”. The mean r 2 was adopted to represent the average LD for each group and LD decay figures were drawn using R [ 27 ].
2.6. Selective Sweep Analysis
The population of genetic summary statistics (θπ, θw, Tajima’s D and Fst; Supplementary Materials ) was calculated for the two populations. The following criteria were used to identify candidate genes in each of the two pairwise comparisons: Fst values >95% of the population pairwise distribution; θπ and θw higher in A and < 10% of the B population distribution; negative Tajima’s D values in the A/B population. The mean log-likelihood-ratio test statistic assessed significance. The heterozygosity log 2 (θπH/θπD ratio) was calculated over a 100 kb sliding window with a step of 20 kb. All annotated genes that overlapped with sweep windows or their flanking windows (20 kb up- and downstream of the sweep region) were defined as candidate genes. GO categories [ 28 ] assessed gene family enrichment across subsets of genes under selection and invariant genes. The analysis first mapped all the candidate genes to the related term in the Gene Ontology database ( http://www.geneontology.org/ accessed on 13 August 2020), calculated the number of target genes mapped to each term, and then used hypergeometric tests to identify the GO entries that were significantly enriched in the candidate target genes compared with the whole reference genome, in which the p values were corrected using the Benjamini–Hochberg method [ 29 ].
3.1. Sequencing and Variation
This study selected eight tigers with two distinct geographic distributions, including five Amur tigers and three South China tigers ( Figure 1 a). We sequenced these tigers to an average of >25× genome coverage depth using the Illumina HiSeq PE150 to generate 150 bp paired-end reads. The raw reads were subjected to a series of quality control procedures ( Supplementary Materials ), and then the filtered high-quality reads were mapped back to the Amur tiger genome (GCA_000464555.1).

Distribution and variation analysis of tigers in China. ( a ) Distribution of tigers (the distribution of the South China tiger was the last result of a survey by the National Forestry and Grassland Administration; the South China tiger is now considered extinct in the wild). ( b ) SNP location and distribution. ( c ) Mutation type and quantity distribution of SNP. ( d ) InDels length distribution in genome. ( e ) CNV location and distribution.
Resequencing of the tiger yielded 648 billion 150 bp paired-end reads, which comprised 647 Gb of high-quality raw data ( Supplementary Table S2 ). Sequence reads were aligned to the Amur tiger reference genome (SRA074975) using BWA software [ 21 ]. The mapping rate in different individuals varied from 97.55% to 98.16%, averaging 97.96%. The average final effective mapping depth achieved was ~28× per individual, ranging from 25× to 30×.
Using a conservative quality filter pipeline (see Materials and Methods Section 2.3 ), we identified 6,338,767 SNPs genome-wide in two subspecies ( Supplementary Table S3 ) based on comparisons to the reference genome. Of the 6.3 million high-quality SNPs, most (66.3%) were located in intergenic regions, with an average of 31.72% located in coding sequences ( Supplementary Table S3 ; Figure 1 b,c). Coding regions displayed lower diversity levels relative to intron and UTR sequences. There were 25,148 synonymous and 18,534 non-synonymous SNPs among the coding areas, resulting in a non-synonymous-to-synonymous substitution ratio of 0.74. Moreover, we investigated the SNPs that are likely to significantly impact gene function, including mutations that lead to stop gain, stop loss, and altered splicing.
In addition to SNPs, we identified 1,727,980 small insertions and deletions (InDels) in the Amur tiger and South China tiger. The InDels ranged from 1–200 bp in length ( Supplementary Tables S4 and S5 ). Most InDels (99.58%) were small (1–6 bp), with only 0.42% greater than 20 bp in length ( Figure 1 d; Supplementary Table S5 ). The majority (64.76%) of the InDels were located in intergenic regions, and 32.34% were located in coding sequences, among which only 0.38% were in-frame, three bp InDels. These genes with large effect mutations might be important in the functional evolution of tiger genes. This dataset provides a set of molecular markers that could be used to identify evolutionary traits in related genes [ 1 , 30 , 31 , 32 ].
268,187 copy number variations (CNVs) were identified ( Figure 1 e; Supplementary Table S6 ). The number of SNPs was higher in the Amur tiger than in the South China tiger ( Supplementary Table S3 ). As a result, the sample sizes of the two subspecies are not equal. After the number of individuals was normalized, there were about 780,000 SNPs in the South China tigers, and about 1.86 million in the Amur tigers, which is around 2.4 times as much as the former. Therefore, it can be speculated that the South China tiger has lower polymorphism. Since the South China tiger is extinct in the wild, all of the captive South China tigers are the offspring of six individuals captured in the mid-20th century. This led to a higher degree of inbreeding, decreasing genetic heterogeneity and genetic diversity.
3.2. The Divergence between the Amur Tiger and the South China Tiger
Recent studies [ 1 , 30 , 31 , 32 ] proposed that Amur tigers came from the South China tiger. To observe the divergence between the Amur tiger and the South China tiger at the genomic level, we constructed a rooted neighbor-joining phylogenetic tree based on 6,338,767 high-quality SNPs ( Figure 2 a). The Amur tiger and the South China tiger were separated into two large clades. In addition, we used mitochondrial genomes to construct phylogenetic trees for seven tiger subspecies and Felis catus, which were divided into two large clades. The phylogenetic tree produced from eight species proves that the Amur tiger and the Bengal tiger are all differentiated from the South China tiger ( Figure 2 b), which is consistent with the findings of Junsong Shi [ 32 ].

Divergence between the Amur tiger and the South China tiger. ( a ) Phylogenetic relationship analysis of eight tiger breeds. ( b ) Phylogenetic relationship among the Panthera tigris . ( c ) Three-dimensional principal component analysis. ( d ) Population structure. Each column in the picture represents an individual, and the length of the different color fragments represents the proportion of an ancestor in the individual genome. ( e ) Decay of linkage disequilibrium (H: the South China tiger; D: the Amur tiger.).
The principal component analysis ( Figure 2 c) shows that the South China tiger separated on main component 1, followed by the Amur tiger on main component 2. We performed population structure analysis using the software PLINK, with K changing progressively from 2 to 5 and dividing the tigers into two subspecies. The results of the population structure analysis were similar to that of the evolutionary tree and principal component analysis, as can be seen from Figure 2 d; when K = 2, it was evident that the eight individuals were divided into two groups, which was consistent with the basic information of the sample collected in this experiment. There was a small gene exchange between the Amur tiger and the South China tiger. When K = 3, five Amur tigers were divided into four groups, which was consistent with the genetic distance of two Amur tigers in the principal component analysis. When K = 4, the five Amur tigers were distinct. When K = 5, the three South China tigers were also separate. This shows that there is a specific genetic distance between each individual. The small gene exchange means that both do not have complete geographical isolation. All the above analyses consistently showed that they have a closer phylogenetic relationship.
We estimated the population diversity parameters, π and θω, and found that the overall nucleotide diversity in the Amur tiger was higher than that in the South China tiger ( Table 1 ). The linkage disequilibrium (LD) distance of the two subspecies was almost similar (between 54.5 kb and 48.3 kb) ( Figure 2 e). The LD was increased markedly in the South China tiger compared with the Amur tiger, with the LD reaching half of its maximum value when the r 2 value of the Amur tiger is about 0.4 and the South China tiger is about 0.6. The South China tiger population has a high degree of LD compared to the Amur tiger population.
Summary SNP statistics in Panthera tigris genotypes.
Genome-Wide | SNP no. | θπ (10–3) | θω (10–3) | Tajima’s D | Non-Syn SNPs | Syn SNPs | Non-Syn/Syn |
---|---|---|---|---|---|---|---|
5,579,192 | 0.876 | 0.812 | 0.423 | 16,099 | 21,948 | 0.734 | |
3,885,781 | 0.563 | 0.526 | 0.336 | 11,106 | 15,268 | 0.727 |
3.3. Screening and Annotation of Selective Sweeps based on SNP
We explored the genomic regions with high divergence to identify essential genes that possibly govern physiological traits, such as environmental adaptions. We calculated the fixation index (F st ) [ 33 ] between subspecies, and the genomic regions with F st values in the top 5% were considered highly differentiated ( Figure 3 a; Supplementary Table S7 ).

Screening of selective sweeps. ( a ) Genome–wide distribution of F st between the Amur and the South China tiger base on SNP. The horizontal dashed line indicates the threshold defining the selective sweeps (F st ≥ 0.59). ( b ) The θπ distribution. ( c , d ) Identification of genomic regions with strong selective sweep signals in Amur tiger and South China tiger. Data points located to the right of the vertical lines (corresponding to 5% right tail of the empirical log 2 (θπ ratio) distribution) and above the horizontal line (5% right tail of the empirical F st distribution) where identified as selected regions (red points). ( e ) Selection analyses identified the selection signal based on V st method. A dashed horizontal line indicates the cut−off (V st > 0.439) used for extracting outliers.
We performed a selective sweep analysis over the whole genome to identify candidate genes under positive selection in different tiger subpopulations. We scanned the genome in 40 kb sliding windows with a step size of 20 kb. We calculated the reduction of diversity based on the population differentiation F st and π values ( Supplementary Tables S8 and S9 ) in the Amur tiger and the South China tiger, respectively ( Figure 3 b–d). The regions in the top 5% were considered as selective sweeps. Fisher’s exact test obtained the significance of all statistics. In total, we identified 702 and 414 candidate genes in the Amur tiger and the South China tiger, respectively ( Supplementary Tables S10 and S11 ).
We performed a Gene Ontology (GO) enrichment analysis of the gene sets in sweep regions of the Amur tiger and the South China tiger. We found that genes with essential biological functions, such as reproduction, carnivorous diet, metabolism, and disease were significantly enriched in the Amur tiger ( Supplementary Tables S12 and S13 ). For example, a set of key terms was found related to the process of mating and reproduction, such as spermatid development (GO:0007286), mating-type factor pheromone receptor activity (GO:0004932), and hormone activity (GO:0005179). Moreover, other enriched functions include regulation of response to stress (GO:0080134), regulation of defense response (GO:0031347), immune response (GO:0006955), cardiovascular system development (GO:0072358), as well as many GO clusters in the regulation of blood pressure vasodilation (GO:0008217, GO:0042311). All functional terms mentioned above had a significant enrichment score ( p -value < 0.05) after considering multiple testing errors using the Bonferroni method. Besides, categories associated with muscle function, such as smooth muscle contraction (GO:0006939), muscle organ development (GO:0007517) and muscle structure development (GO:0061061), and sensory organs (GO:0050909, GO:0007600, GO:0007601, GO:0007608) were also characterized. Then, we performed KEGG pathway analysis on the genes in these regions, and 220 pathways were obtained; some KEGG pathways are shown in Supplementary Tables S14 and S15 . These pathways are mainly metabolized, e.g., glycerolipid metabolism, tyrosine metabolism, nitrogen metabolism, tryptophan metabolism, pyruvate metabolism, and glycerophospholipid metabolism. These amino acid metabolic signals are associated with a compulsive carnivorous diet. Signal transduction occurs in many pathways, e.g., HIF-1 signaling pathway, cGMP-PKG signaling pathway, cAMP signaling pathway, TNF signaling pathway, calcium signaling pathway, insulin signaling pathway. These pathways are related to sight and smell, which are important for the ownership of territory, mating, and hunting. Synthesis of hormones includes N-Glycan biosynthesis, fatty acid biosynthesis, steroid hormone biosynthesis, and primary bile acid biosynthesis. The diseases include legionellosis, toxoplasmosis, pertussis, leishmaniasis, dilated cardiomyopathy, and malaria. The significant enrichment pathway ( p < 0.05) is shown in Table 2 .
KEGG pathway in Amur tiger ( p < 0.05).
Species | Term | Database | ID | Gene Amount | -Value |
---|---|---|---|---|---|
Amur tiger | Ribosome | KEGG PATHWAY | fca03010 | 18 | 0.0287 |
Through studying the selected regions, we found that both tigers have a lot in common with regard to the enrichment of gene function and metabolic pathway analysis, such as in the carnivorous diet, muscular strength, metabolic diseases, etc. Tigers are located at the top of the food chain in mammals, thus they have high vigilance, high-intensity energy metabolism, and muscle strength. Additionally, their keen senses of smell, hearing, and sharp instincts alone are enough to make them adapt to a complex ecological environment, which corresponds to their rank in the niche.
In the two subpopulations, we observed a consistently strong signal of positive selection on chromosome 10 NW_006712238.1, which harbors a Toll-like receptor 4 ( TLR4 ) gene ( Table 3 ). The TLR4 gene was found to be involved in many metabolic pathways related to legionellosis, toxoplasmosis, malaria, amoebiasis, and leishmaniasis. The protein encoded by the TLR4 gene is a member of the Toll-like receptor ( TLR ) family, which plays a fundamental role in pathogen identification and innate immune activation. Its activation leads to cell signaling pathways within the NF-κB and the production of inflammatory cytokines, which activate the innate immune system [ 34 ]. Genetic diagnosis can be used to study the mechanism of the parasite and perform early prevention and timely diagnosis.
KEGG pathway and genes related to the disease.
KEGG ID | Description | Gene Name |
---|---|---|
fca05134 | Legionellosis | TLR4, CASP3, UBE2E2, LOC102967742, TRRAP, CNTN1 |
fca05145 | Toxoplasmosis | LY96, TLR4, CASP3, SFXN1, MAPK8, MAP3K7, IFNGR1, ITGB1 |
fca05133 | Pertussis | LY96, TLR4, CASP3, MAPK8, LOC102967742, ITGB1 |
fca05140 | Leishmaniasis | SFXN1, MAP3K7, IFNGR1, TLR4, ITGB1 |
fca05414 | Dilated cardiomyopathy | LOC102950759, SFXN1, CACNA1D, MYL3, TPM4, ITGB1 |
fca05144 | Malaria | SFXN1, TLR4, HGF, GYPC |
fca05014 | Amyotrophic lateral sclerosis (ALS) | GRIA4, LOC102967742, CASP3 |
fca05016 | Huntington’s disease | CLTCL1, LOC102962163, LOC102957675, CLTC, CASP3, NDUFA4, AP2A2, CREB3L2 |
fca05162 | Measles | ADAR, TNFAIP3, IFNGR1, TLR4, MAP3K7 |
fca05168 | Herpes simplex infection | TRAF5, CASP3, MAPK8, MAP3K7, SRSF6, IFNGR1, CCPG1 |
fca05164 | Influenza A | ADAR, TMPRSS2, TLR4, MAPK8, LOC102967742, IFNGR1 |
fca05010 | Alzheimer’s disease | YPEL2, ATP2A3, CACNA1D, LOC102953458, CASP3, NDUFA4 |
fca05012 | Parkinson’s disease | CASP3, NDUFA4, GPR37, LOC102962163, UCHL1 |
fca05152 | Tuberculosis | TLR4, CASP3, SFXN1, FARSB, MAPK8, IFNGR1 |
fca05146 | Amoebiasis | SFXN1, TLR4, CASP3 |
fca05416 | Viral myocarditis | CASP3 |
In addition to TLR4 , we also characterized some of the two subspecies’ candidate genes with a significantly high window Fst and heterozygosity ratio (HP) that are functionally plausible for adaptation in the wild. For example, these genes include: (1) TEX22 , FOXO1 , FBXL17 , MCTP1 , and ASB2 associated with smell; (2) FAM189A1 and PDE6H associated with vision [ 35 , 36 ]; (3) ADAMTSL3 , FAM189A1 , FAM135B , DPP10 and FBXL13 involved in muscle development [ 37 ]; and (4) EFHC2 , CDH13 , DNM3 , RAD51D , CDH13 , GPC6 , ROBO1 , LECT2 and PLB1 for regulating production [ 23 , 38 , 39 , 40 , 41 , 42 , 43 , 44 ].
3.4. Selective Elimination Analysis based on CNV
The analysis of CNV in the selected region of the tiger’s genome was identified by selecting the signal. Figure 3 e shows the distribution of V st of the Amur tiger population and the South China tiger population in the genome (0 ≤ Vst ≤ 1, the closer the V st approaches 1, the more obvious the differentiation is between subpopulations; Supplementary Materials ). The V st threshold in the 5% selected area is 0.493. Accordingly, 782 selected regions were identified in this study, with a total length of 3.9 Mb and an average length of about 4.9 kb, containing 94 genes ( Supplementary Table S16 ). As can be seen from Figure 3 e, there are more outlier sites between the Amur tiger and the South China tiger. Many chromosome regions have large V st values, and the largest V st value is 0.999, which is located in the chromosome NW_006712148.1 region, and there are seven CNVs in the selected region.
To assess possible gene functions targeted by both the Amur tiger and the South China tiger, we performed a Gene Ontology (GO) enrichment analysis of the gene sets in sweep regions of the two subpopulations ( Supplementary Table S17 ). The results showed many irritability-related functional terms over-represented in their overlapping candidate genes ( Supplementary Table S18 ). For example, a set of key terms was found related to stimulus–response, such as cellular response (GO:0051716, GO:0051716). Categories associated with signal receptor activity, such as signal transduction (GO:0007165), G-protein-coupled receptor signaling (GO:0007186), and cell communication (GO:0007154). Moreover, other enriched functions include biological regulation (GO:0065007), integral to membrane functions (GO:0016021), and receptor activity (GO:0004872). All functional terms mentioned above had a significant enrichment score ( p < 0.05) after considering multiple testing errors (by the hypergeometric test/Fisher’s exact test).
The genes in these selected regions were analyzed via the KEGG pathway, and 63 biochemical pathways were obtained ( Supplementary Table S19 ). The significant enrichment ( p < 0.05) pathway is olfactory transduction. In addition, these pathways are mainly metabolized, as found with phenylpropane metabolism, phenylalanine metabolism, β-alanine metabolism, tyrosine metabolism, retinol metabolism, and olfactory transduction. Interestingly, some disease pathways were also found, such as autoimmune thyroid disease, type I diabetes mellitus, type II diabetes mellitus, Epstein–Barr virus infection, legionellosis, viral myocarditis, toxoplasmosis, measles, systemic lupus erythematosus, influenza A, Huntington’s disease, HTLV-I infection, and so on. These are consistent with the results of the KEGG metabolic pathway based on SNP.
3.5. The Differentiation Mechanism of Tiger Subspecies
Bergmann’s rule states that endothermic animal subspecies living in colder climates have larger bodies than that of the subspecies living in warmer climates [ 45 ]. Individuals with larger bodies are better suited for colder climates because larger bodies produce more heat due to having more cells, and have a smaller surface area compared to smaller individuals, which reduces heat loss. Compared with the South China tiger, the Amur tiger is relatively large in body size, longer in fur, thinner in pattern, and lighter in color. Additionally, it has a very thick white coat around its neck to accommodate the cold weather; our data also provided new evidence of how the Amur tiger adapts to the cold. By comparing our candidate gene list with those from the Amur tiger, we found PRKG1 as a consistent signal in response to cold adaptation identified among independent approaches. Our results indicated that PRKG1 was under positive selection in Amur tiger populations. The PRKG1 gene can be involved in smooth muscle contraction [ 46 , 47 ] and the maintenance of normal blood pressure [ 48 , 49 ], which can help to avoid heat loss and is also important for body temperature regulation. The PRKG1 protein is best known for its cardiovascular and neuronal functions and it is expressed in cerebellar Purkinje cells, hippocampal neurons, and the lateral amygdala, as well as platelets. In mammals, PRKG1 -phosphorylated proteins are known to regulate cardiac function, gene expression, feedback of the NO-signaling pathway, and processes in the central nervous system including axon guidance, hippocampal and cerebellar learning, circadian rhythm, and nociception [ 50 ].
Nevertheless, it is intriguing that other possible candidate genes of cold adaptations, such as FOXO1 and TPM4 , more often exhibit lineage-specific signals. The mammalian body always works to remain in homeostasis. One form of homeostasis is thermoregulation. The normal temperature of the tiger is between 37.5 °C and 39.5 °C. Stress from extreme external temperatures can cause the body to shut down [ 51 ]. FOXO proteins are major targets of insulin action, and FOXO1 mediates the effects of insulin on hepatic glucose metabolism [ 52 ]. FOXO1 is significantly expressed in the gene of the Amur tiger and regulates insulin secretion, which acts on carbohydrate metabolism and, thus, increases energy production [ 53 ]. Therefore, we believe that FOXO1 is closely related to body temperature maintenance. These cold temperatures commonly result in mortality. Tigers have adapted to living in these extreme climates primarily through autoregulation. Evidence suggests that tigers may have lived in the ice age and then spread to Africa and the Americas, suggesting that the tigers were adaptable enough to live in various climatic conditions early on. This is supported in the variability selection hypothesis, which says that mammalian adaptability came from environmental change over the long term [ 54 , 55 ].
4. Discussion
The evolution of the tiger is known from several pieces of evidence of fossil and phylogenetic analysis. The oldest fossil, called Felis palaeosinensis , was found in northern China and Java [ 56 ]. It is thought to date from the end of the Pliocene and the beginning of the Pleistocene, being more than two million years old [ 57 ]. Collier and O’Brien supported this hypothesis by showing that the tiger diverged from other Panthera species up to two million years ago, before the divergence of the lion, leopard, and jaguar [ 58 ]. The center of evolution is thought to be in northern China [ 59 ]. The Amur tiger and the South China tiger belong to the genus panther in the cat family. Both have the same adaptive ability, which is consistent with the carnivorous and muscular strength of the carnivorous panthers. The Amur tiger and the South China tiger genomes show similar repeat compositions and high genomic synteny, indicating strong genomic conservations in Felidae [ 20 ].
Geographical isolation promotes the differentiation of species or subspecies. Tigers in the Russian far east are larger than those in Sudan Island, which may be due to growth hormones stimulated by cold weather, and may also be responsible for the divergence between the South China tigers and the Amur tigers. To adapt to the cold climate, the species can minimize the loss of unit energy by increasing the surface area, as the larger weight can store more energy and fat to resist the cold. Long-term adaptation can lead to some genes being fixed in this trait. Selective elimination occurs when a rare or previously nonexistent allele (relative to individuals of other populations) increases rapidly under the action of natural selection. Through gene selection analysis, we found that PRKG1 in the genome of the Amur tiger was involved in temperature regulation in a cold environment. FOXO1 and TPM4 regulate body temperature to keep it constant, providing new insights for how Amur tigers adapt to cold climates. The body-temperature-related genes FOXO1 and TPM4 appear to have been strongly selected in the Amur tiger. Amur tigers live in northeast China and the far east of Russia, where in winter it becomes covered in snow and the average temperature is below −20 °C. The cold environment stimulates the expression of genes related to body temperature regulation in Amur tigers. Recent studies have also shown that there are genes adapted to the cold environment in the Amur tiger genes [ 60 ]. The expression of positive selection genes is closely related to energy metabolism and muscle contraction, which may be closely associated with the habitat environment of the Amur tiger. Adaptation to a cold climate may be one of the reasons for the differentiation between the Amur tigers and the South China tigers, similar to the differentiation between the Polar bear ( Ursus maritimus ) and the Brown bear ( U. arctos ) [ 61 ].
The LD of the South China tiger was significantly higher than that of the Amur tiger, which may be related to inbreeding and the effective population size of the South China tiger. About 66 years ago, the effective population of the South China tiger was lost [ 15 ]. Inbreeding has taken place since captivity in 1963; continuous inbreeding has also led to some problems that are not conducive to population reproduction [ 8 , 15 , 62 ]. However, the inbreeding of Amur tigers in captivity is low [ 63 ]. Principal component analysis and population structure analysis also showed that the South China tiger samples were closely related, whereas the Amur tiger samples were distantly related. Of course, the error caused by the small sample size cannot be ruled out, but this result should also be paid attention to by managers because closely related groups are likely to lead to inbreeding.
The research shows that scientific management could reduce the risk of inbreeding [ 62 ]. However, recent studies have claimed that scientific management can only solve the current inbreeding and cannot solve the long-term problem [ 15 ]; therefore, the scientists suggest that gene rescue should be used to reduce South China tigers’ inbreeding [ 8 ]. Our study found genetic differences in environmental adaptation between the Amur tiger and the South China tiger. The Amur tiger is genetically adapted to the cold environment, which is contrary to the warm environment suitable for the South China tiger. These genes might become novel genetic loads for the South China tiger gene pool given they are introduced. We suggest that the recovery of the South China tiger population using gene rescue should be carefully selected for homologous species, especially for environmental adaptation.
5. Conclusions
We used whole-genome sequencing technology to compare and analyze the Amur tiger and South China tiger genomes. The results showed that: 1. The Amur tiger and the South China tiger are separated into two large clades. 2. The South China tiger population has a high degree of LD compared to the Amur tiger population. 3. We identified functional genes and regulatory pathways related to reproduction, disease, predation, and metabolism and characterized functional genes related to survival in the wild. PRKG1 is involved in temperature regulation in a cold climate. FOXO1 and TPM4 regulate body temperature to keep it constant.
Acknowledgments
We thank the Northeast Tiger Park and Shanghai Zoo for providing samples in this study, and we thank the instructors.
Supplementary Materials
The following supporting information can be downloaded at: https://www.mdpi.com/article/10.3390/ani12141817/s1 , Tables S1–S19 (Table S1. Sample information; Table S2. Data output report and quality statistics; Table S3. Distribution of SNPs within genomic regions of two breeds; Table S4. Genetic distribution of detected InDels; Table S5. InDels length distribution in CDS and genome; Table S6. CNV statistical results in each individual genome; Table S7. Genome-wild distribution of Fst between Amur and South China tiger; Table S8. Genome-wide distribution of θπ in Amur tiger; Table S9. Genome-wild distribution of θπ of South china tiger; Table S10. The region and genes of amur tiger in significantly selected region; Table S11. Selection region and candidate genes in south China tiger; Table S12. GO enrichment analysis in the Amur tiger; Table S13. GO significant enrichment analysis in the South China tiger; Table S14. KEGG pathway in Amur tiger; Table S15. KEGG pathway in South China tiger; Table S16. Significant enrichment genes in the selective signal region based on CNV; Table S17. The enriched ontology terms of overlap gene in selection regions base on CNV; Table S18. The gene information both in Amur tiger and South China tiger in selected region; Table S19. KEGG pathway of overlap gene in selection regions base on CNV); Word S1. Quality control procedures of details.
Funding Statement
This work was supported by the Habitat Restoration Pilot Program II of Amur Tiger and Amur Leopard Project; and the Fundamental Research Funds for the Central Universities [2572016AA45].
Author Contributions
H.D. is responsible for paper writing and data processing; Q.L. and J.Y. are accountable for data processing and manuscript modification; M.Z. provided funds, research design, and manuscript modification. All authors have read and agreed to the published version of the manuscript.
Institutional Review Board Statement
This study’s sample collection and experiment were reviewed and approved by the Ethics Committee of Northeast Forestry University.
Data Availability Statement
Conflicts of interest.
The authors declare that they have no known competing financial interests or personal relationships that could have appeared to influence the work reported in this paper.
Publisher’s Note: MDPI stays neutral with regard to jurisdictional claims in published maps and institutional affiliations.
- IAS Preparation
- UPSC Preparation Strategy
- Tiger Conservation India
Tiger Conservation in India
In July 2020, the Ministry of Environment released the Tiger Census Report on the eve of Global Tiger Day (29th July). India has 70 percent of the world’s tiger population. It has been announced that Conservation Assured | Tiger Standards (CA|TS) framework will be extended to all 50 Tiger Reserves of India.
As per the 4th All India Tiger Estimation (AITE), there are 3,167 tigers in India. Get more details on its conservation and check the tiger reserves in India map for quick revision for UPSC 2023 .
Tiger Conservation Latest News
The tiger population “part report” was published to mark the 50th anniversary of Project Tiger in April. Read more on this development in the link here .
RSTV – Big Picture: Importance of Tiger Conservation – Aspirants can check the linked article to get more points on the topic from Rajya Sabha TV discussion (Aug 2020).
|
Table of contents:
Tiger Symbol for Power and Energy
Tiger is the National Animal of India and also has a significant position in Indian culture. Tiger usually symbolizes Power and enormous Energy.
Some interesting facts related to Tigers in India:
- Nagpur is also known as the ‘Tiger Capital’ of India
- There are 13 tiger reserves in Vidharbha (including the Nagpur division of eastern) alone.
- The national parks around Nagpur include Umred Karhandla Wildlife Sanctuary, Pench National Park, Nagzira-Navegaon Tiger Reserve, Tadoba-Andhari Tiger Reserve, Melghat Tiger Reserve, and Bor Tiger Reserve.
- The Climatic condition of this forested region is very appropriate for tiger conservation.
- First white tiger sanctuary – Madhya Pradesh (The maiden ‘White Tiger Safari’ was inaugurated in 2016.)
- Tiger IUCN Status – Endangered
Get more interesting facts about the National Animal of India in the linked article.
The list of tiger reserves in India is given below:
1 | Andhra Pradesh | Nagarjunsagar Srisailam |
2 | Arunachal Pradesh | Namdapha National Park |
3 | Arunachal Pradesh | Kamlang Tiger Reserve |
4 | Arunachal Pradesh | Pakke Tiger Reserve |
5 | Assam | Manas Tiger Reserve |
6 | Assam | Nameri National Park |
7 | Assam | Orang Tiger Reserve |
8 | Assam | Kaziranga National Park |
9 | Bihar | Valmiki National Park |
10 | Chhattisgarh | Udanti-Sitanadi Wildlife Sanctuary |
11 | Chhattisgarh | Achanakmar Wildlife Sanctuary |
12 | Chhattisgarh | Indravati Tiger Reserve |
13 | Jharkhand | Palamau Tiger Reserve |
14 | Karnataka | Bandipur Tiger Reserve |
15 | Karnataka | Bhadra Wildlife Sanctuary |
16 | Karnataka | Dandeli-Anshi Tiger Reserve |
17 | Karnataka | Nagarahole National Park |
18 | Karnataka | Biligiri Ranganatha Temple Tiger reserve |
19 | Kerala | Periyar Tiger reserve |
20 | Kerala | Parambikulam Tiger reserve |
21 | Madhya Pradesh | Kanha Tiger reserve |
22 | Madhya Pradesh | Pench Tiger reserve |
23 | Madhya Pradesh | Bandhavgarh Tiger reserve |
24 | Madhya Pradesh | Panna Tiger reserve |
25 | Madhya Pradesh | Satpura Tiger reserve |
26 | Madhya Pradesh | Sanjay-Dubri Tiger reserve |
27 | Maharashtra | Melghat Tiger reserve |
28 | Maharashtra | Tadoba-Andhari Tiger Reserve |
29 | Maharashtra | Pench Tiger Reserve |
30 | Maharashtra | Sahyadri Tiger Reserve |
31 | Maharashtra | Nagzira Tiger Reserve |
32 | Maharashtra | Bor Tiger Reserve |
33 | Mizoram | Dampa Tiger Reserve |
34 | Odisha | Similipal Tiger Reserve |
35 | Odisha | Satkosia Tiger Reserve |
36 | Rajasthan | |
37 | Rajasthan | Sariska Tiger Reserve |
38 | Rajasthan | Mukandra Hills Tiger Reserve |
39 | Tamil Nadu | Kalakad-Mundanthurai Tiger Reserve |
40 | Tamil Nadu | Anamalai Tiger Reserve (Indira Gandhi Wildlife Sanctuary and National Park) |
41 | Tamil Nadu | Mudumalai Tiger Reserve |
42 | Tamil Nadu | Sathyamangalam Tiger Reserve |
43 | Telangana | Kawal Tiger Reserve |
44 | Telangana | Amrabad Tiger Reserve |
45 | Uttar Pradesh | Dudhwa Tiger Reserve |
46 | Uttar Pradesh | Pilibhit Tiger Reserve |
47 | Uttar Pradesh | Amangarh Tiger Reserve (buffer zone of Corbett Tiger Reserve) |
48 | Uttarakhand | |
49 | Uttarakhand | Rajaji Tiger Reserve |
50 | West Bengal | Sunderban National Park |
51 | West Bengal | Buxa Tiger Reserve |
Tiger Conservation in India Notes:- Download PDF Here
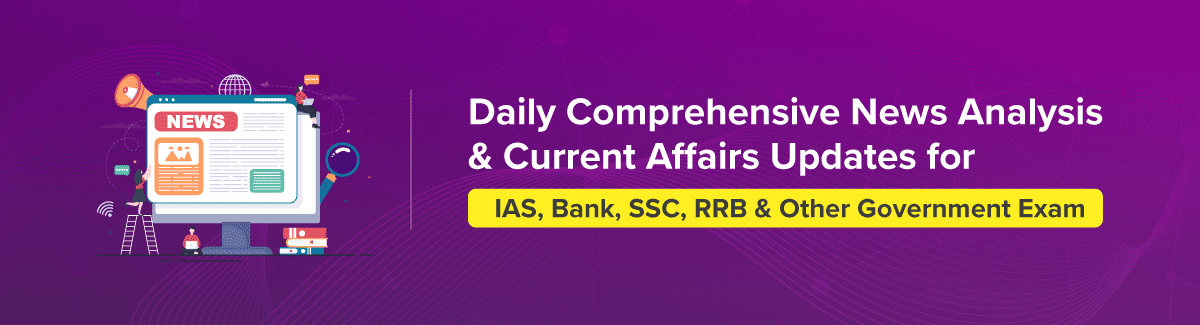
Tiger Reserves in India Map
The geographical location of Tiger Reserves in India [Map] is shown below:
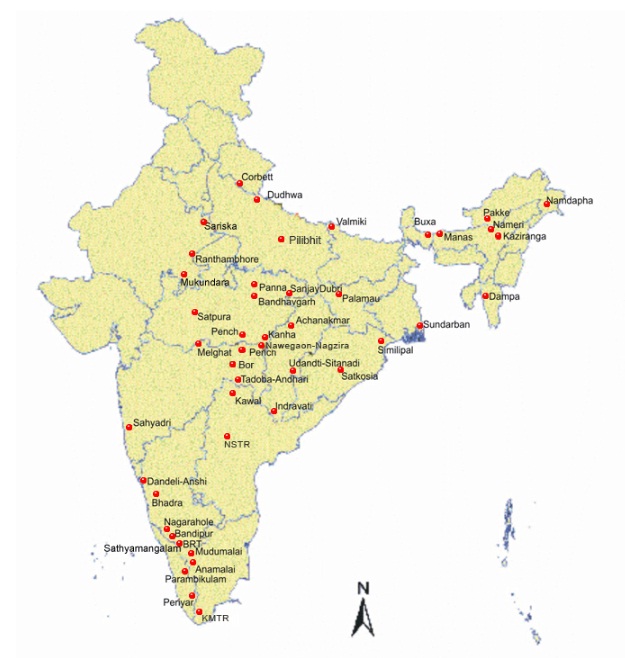
As seen on the map, it is evident that the tiger reserves in India are mostly in the Central and Eastern parts. It is necessary on the part of the inhabitants to help the government to establish more Tiger reserves in the Western areas as well.
Related Links:
New Tiger Reserves in India
Recently, the National Tiger Conservation Authority has agreed to the formation of four new tiger reserves in:
- Sunabeda (Odisha),
- Ratapani (Madhya Pradesh)
- Orang (Assam)
- Guru Ghasidas (Chhattisgarh)
The respective state governments have been directed to send the proposal for announcing Suhelwa(Uttar Pradesh), Cauvery MM Hills (Karnataka), Mhadei(Goa), Srivilliputhur Grizzled Giant Squirrel (Tamil Nadu) and Dibang (Arunachal Pradesh) as tiger reserves.
Critical Tiger Habitat
The Critical Tiger Habitats (CTHs) as per Wildlife Protection Act, 1972, are core areas of the tiger reserves meant to be “kept inviolate for the purpose of tiger conservation.” For recognition of critical tiger habitat, an expert committee is constituted and the final decision is notified by the state government.
In Forest Rights Act, 2006 , there is a mention of ‘Critical Wildlife Habitats’ which are part of reserves and sanctuaries meant to be kept inviolate for wildlife conservation (not just tigers). In the case of Critical Wildlife Habitats, Gram Sabhas and affected stakeholders have to give consent for the notification.
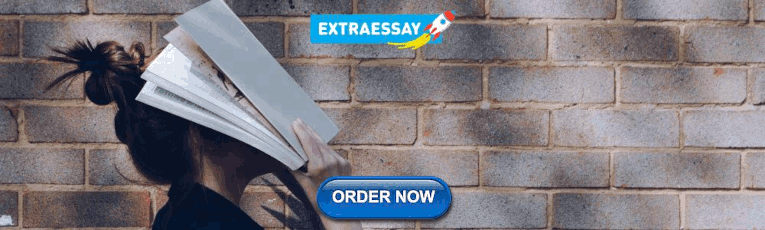
Threats to Tiger Population

The Tigers are seen largely in the tropical forests of Asia. The recent reports generated by different international organizations show the tiger population is decreasing. According to the IUCN Red list , the tiger is listed as an endangered animal. The major threat faced by this species are Poaching, destruction of habitat, insufficient prey, etc. the tigers are killed for skins, bones, and meat.
The list of major threats to the Tiger Population are:
- Man- animal conflict
- Hunting, poaching and illegal trade
- Habitat and loss of prey species
Man-animal conflict
Due to anthropogenic activities, the tiger population has lost its habitat. The loss of habitat resulted in the reduction of their prey species. They started coming out of the forest and come to the village in search of prey.
Tiger those who came in search of its prey they attacked domestic animals, and human beings. In vengeance, tigers are often killed by angry human beings.
Read in detail about Human-Wildlife Conflict from the linked article.
Hunting, illegal trade and poaching
Hunting of tigers is a huge issue faced by the tiger population from ancient times. Hunting stands as a symbol of status. The people used the bones, teeth of the tiger for commercial purposes which can also be named as illegal trade.
The body parts are used for medicinal purposes also. This has caused the utmost decline in tiger populations since 1930. Poaching is the next threat to the leftover tiger population.
Anthropogenic Activities
The anthropogenic destructive activities that have caused the devastation of habitat and prey species are the main long-term threats to the continuation of the declining tiger population in the country.
Man and animals compete for finding their habitat. Tigers need large territories. Along with the habitat, tigers have also experienced a severe loss of prey species populations such as antelopes and deer.
The man has acquired the forest land for human settlements and industrial activities. This has ultimately resulted in complete destruction of animal habitats.
The same affected the climatic condition, harmony, and poses a threat to the existence of human beings. The Ken-Betwa River interlinking project which intends to irrigate the drought-hit Bundelkhand region is also a reason for the declining Tiger population in India. According to the wildlife experts, the Panna Tiger Reserve which is located near this place will have a greater impact due to the project.
Rise in Population of Tiger
Recent years have seen a rise in the population of wild lions and tigers in India. The government has initiated many programs related to the conservation of the Tiger population in the country.
According to the World Wildlife Fund and the Global Tiger Forum, the figure of wild tigers has gone up to 3,890 which earlier was 3200.
The wildlife policy of India embraces conservation and protection through the provision of national parks and sanctuaries. This has assisted their sustainable growth and a drastic declining man-animal conflict. A proactive judiciary has shaped a well-built restriction against poachers.
India is now home to 70% of the total Global Tiger Population. The various strategies that are planned for implementation include:
- Tiger reintroduction
- Landscape conservation, and habitat management
- Scrutinizing protocols, strategies for anti-poaching
- Resource Mobilization, and
- Modern equipment and technology for monitoring.
The anti-poaching strategies are also planned for implementation. The latest censuses show an augment in the number of tigers across the country in different National Parks which is evidence that preservation efforts do have an optimistic impact on the environment and wildlife.
Reasons for the rise in the population of Tiger
- Establishment of various programs and institutions like TraMCA, Project tiger, Project snow leopards, NGT, aiming at the protection of the tiger and its habitat.
- TraMCA is a joint initiative of India and Bhutan for transboundary biodiversity preservation established in 2008.
- Providing suitable habitat with the availability of prey, water bodies, etc. So that Tiger doesn’t come out which results in man-animal conflict.
- Limiting the tourists to peripheral areas and not core areas where Tiger mate and small cubs generally are taken care of.
- Better trained and efficient Forest administrators and rangers to deal with the issue of hunting and poaching.
- People participate in the conservation of tiger and establishments of Vanya Prani Saathi for the same.
Tiger conservation initiatives in India
A vigorous Tiger population shows the strength of the ecosystem and self-sustenance which are the key objectives of Project Tiger under the National Tiger Conservation Authority.
By launching the Project Tiger , the Government of India has taken a revolutionary initiative for conserving its national animal. It was launched in 1973. It is a 100% Centrally Sponsored Scheme of the Ministry of Environment, Forests and Climate Change.
The Project Tiger is administered by The National Tiger Conservation Authority (NTCA). It is a statutory body of the Ministry with an overall supervisory and coordination part, performing capacities as gave in the Wildlife (Protection) Act, 1972.
Project Tiger
The Project Tiger launched in 1973 is a 100% centrally sponsored scheme. It gives fund help to the ‘tiger range States’, for in-situ conservation of tigers in the chosen tiger reserves. It has put the endangered tiger on a guaranteed path of revival by protecting it from extinction. The Project Tiger aims to promote an exclusive tiger agenda in the core areas of tiger reserves, with inclusive people participation.
The habitats covered under Project tiger are:
- Central India conservation unit
- Shivalik-terai conservation unit
- North East conservation unit
- Sariska conservation unit
- Sunder bans conservation unit
- Eastern Ghats conservation unit
- Western Ghats conservation unit
Objectives of Project Tiger
- To guarantee a viable population of tigers for financial, scientific, aesthetic, social, and ecological values.
- Limit the elements which lead to the reduction of tiger habitat and to tone down them by suitable strategy.
- Site-particular eco-development to decrease the dependency of local individuals and indigenous people on tiger reserve
Core and Buffer Area
- Core Area: The core area has the legal status of a Wildlife Sanctuary or National Park. These areas are free of all forestry operations and human activities.
- Buffer Area: These multi-purpose areas consist of forest and non-land and subjected to conservation-oriented land use.
Benefits of Project Tiger
The Project Tiger has put the tiger on a certain course of revival from the edge of destruction. The population of tigers in the country has been augmented considerably after the establishment of the project. It has contributed to numerous indescribable environmental profits to society. The project has opened a wide door of tourism and thereby employment opportunities. This initiative has brought the participation of local people and their participation has made the habitat to revive.
International Cooperation for Tiger Conservation
- To control the Transboundary illegal trade in wildlife and conservation, India signed a bilateral understanding with Nepal.
- A tiger conservation protocol was signed by India with China
- For the conservation of tigers in the Sunderban region, India has signed a protocol with Bangladesh.
- With Russia, India has constituted a group on tiger and leopard conservation.
- India is a stakeholder of the Convention on International Trade in Endangered Species of Wild Fauna and Flora.
- India doesn’t support captive breeding of tigers.
UPSC previous year Questions
The UPSC has asked a question regarding tiger reserve in UPSC civil service main examination. This shows the importance of the topic. Along with that, we are providing probable UPSC question for practicing.
The issue of tourism in core areas of tiger reserve forests in the country is a subject matter of debate. Critically examine various aspects of this issue, keeping in view relevant recent judicial pronouncements.
Click here to get the UPSC Previous Year Question Paper
Practice questions for UPSC Prelims and Mains Exam
Practice question for UPSC IAS Prelims Exam
Match the following
- Sanjay Dubri A. Uttar Pradesh
- Palamau B. Jharkhand
- Namdapha C. Madhya Pradesh
- Amangarh D. Arunachal Pradesh
- Dampa E. Mizoram
Choose the correct option.
- 1-B, 2-C, 3-D, 4-A, 5-E
- 1-A, 2-B, 3-D, 4-C, 5-E
- 1-C, 2-A, 3-E, 4-B, 5-D
- 1-C, 2-B, 3-D, 4-A, 5-E
Get the previous years’ Topic Wise UPSC Prelims Questions PDF in the linked article.
Practice question for UPSC IAS Mains Exam
With reference to man-animal, what are the socio-economic impacts of man-animal conflicts and the reason for increasing the man-animal conflicts, and what are the steps taken by concerned authorities?
Related Links
Frequently Asked Questions on Tiger Reserves and Conservation in India
Q 1. how many tiger reserves are there in india, q 2. which state has the highest tiger reserves in india.
Ans. As per the Tiger Reserve in India map, Madhya Pradesh has the highest number of Tiger Reserves. There are six tiger reserves in the state:
- Kanha Tiger Reserve
- Pench Tiger Reserve
- Bandhavgarh Tiger Reserve
- Panna Tiger Reserve
- Satpura Tiger Reserve
- Sanjay-Dubri Tiger Reserve
Q 3. Which city is also known as the tiger city in India?
IAS General Studies Notes Links | |
Leave a Comment Cancel reply
Your Mobile number and Email id will not be published. Required fields are marked *
Request OTP on Voice Call
Post My Comment

dear BYJU”s , thank you so much to such wonderful explanation including previous questions and practice questions.

IAS 2024 - Your dream can come true!
Download the ultimate guide to upsc cse preparation, register with byju's & download free pdfs, register with byju's & watch live videos.
Click through the PLOS taxonomy to find articles in your field.
For more information about PLOS Subject Areas, click here .
Loading metrics
Open Access
Peer-reviewed
Research Article
Prioritizing Tiger Conservation through Landscape Genetics and Habitat Linkages
Affiliation Wildlife Institute of India, Chandrabani, Dehradun 248001, India
* E-mail: [email protected]
Affiliations Smithsonian Conservation Biology Institute, National Zoological Park, 3001 Connecticut Avenue, Washington, D.C. 20008, United States of America, Department of Vertebrate Zoology, National Museum of Natural History, Smithsonian Institution, Washington, D.C. 20013, United States of America
Affiliation National Tiger Conservation Authority, Bikaneer House, Shah Jahan Road, New Delhi 110011, India
Affiliation Smithsonian Conservation Biology Institute, National Zoological Park, 3001 Connecticut Avenue, Washington, D.C. 20008, United States of America
- Bibek Yumnam,
- Yadvendradev V. Jhala,
- Qamar Qureshi,
- Jesus E. Maldonado,
- Rajesh Gopal,
- Swati Saini,
- Y. Srinivas,
- Robert C. Fleischer
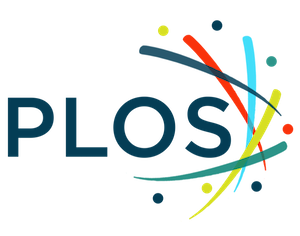
- Published: November 13, 2014
- https://doi.org/10.1371/journal.pone.0111207
- Reader Comments
Even with global support for tiger ( Panthera tigris ) conservation their survival is threatened by poaching, habitat loss and isolation. Currently about 3,000 wild tigers persist in small fragmented populations within seven percent of their historic range. Identifying and securing habitat linkages that connect source populations for maintaining landscape-level gene flow is an important long-term conservation strategy for endangered carnivores. However, habitat corridors that link regional tiger populations are often lost to development projects due to lack of objective evidence on their importance. Here, we use individual based genetic analysis in combination with landscape permeability models to identify and prioritize movement corridors across seven tiger populations within the Central Indian Landscape. By using a panel of 11 microsatellites we identified 169 individual tigers from 587 scat and 17 tissue samples. We detected four genetic clusters within Central India with limited gene flow among three of them. Bayesian and likelihood analyses identified 17 tigers as having recent immigrant ancestry. Spatially explicit tiger occupancy obtained from extensive landscape-scale surveys across 76,913 km 2 of forest habitat was found to be only 21,290 km 2 . After accounting for detection bias, the covariates that best explained tiger occupancy were large, remote, dense forest patches; large ungulate abundance, and low human footprint. We used tiger occupancy probability to parameterize habitat permeability for modeling habitat linkages using least-cost and circuit theory pathway analyses. Pairwise genetic differences ( F ST ) between populations were better explained by modeled linkage costs ( r >0.5, p <0.05) compared to Euclidean distances, which was in consonance with observed habitat fragmentation. The results of our study highlight that many corridors may still be functional as there is evidence of contemporary migration. Conservation efforts should provide legal status to corridors, use smart green infrastructure to mitigate development impacts, and restore habitats where connectivity has been lost.
Citation: Yumnam B, Jhala YV, Qureshi Q, Maldonado JE, Gopal R, Saini S, et al. (2014) Prioritizing Tiger Conservation through Landscape Genetics and Habitat Linkages. PLoS ONE 9(11): e111207. https://doi.org/10.1371/journal.pone.0111207
Editor: Alfred L. Roca, University of Illinois at Urbana-Champaign, United States of America
Received: March 18, 2014; Accepted: September 17, 2014; Published: November 13, 2014
This is an open-access article, free of all copyright, and may be freely reproduced, distributed, transmitted, modified, built upon, or otherwise used by anyone for any lawful purpose. The work is made available under the Creative Commons CC0 public domain dedication.
Data Availability: The authors confirm that all data underlying the findings are fully available without restriction. Data is available from Dryad with the following data identifier: doi: 10.5061/dryad.c7v41.
Funding: Funding provided by the National Tiger Conservation Authority: YVJ QQ, Fulbright Fellowship: BY, Wildlife Institute of India: YVJ BY. The funders had no role in study design, data collection and analysis, decision to publish, or preparation of the manuscript.
Competing interests: Co-author JEM is a PLOS ONE Editorial Board member. This does not alter the authors' adherence to PLOS ONE policies on sharing data and materials.
Introduction
By virtue of being at the top of the food chain, large carnivores occur at low densities, have large home ranges and therefore require vast areas to harbor viable populations [1] . Since historical times, large carnivores have been in conflict with humans for food and resources, often resulting in their demise [2] . Habitat destruction, excessive hunting by humans and the use of body parts for traditional medicine have extirpated many populations [3] , [4] , [5] while severely reducing, fragmenting, and isolating most others to varying degrees [6] , [7] . Small and isolated populations are prone to local extirpation [8] , [9] . Managing such populations in a metapopulation framework [10] , [11] by connecting them through habitat corridors [12] , [13] so that individuals have the opportunity to disperse, establish residency and reproduce, reduces the overall risk of extinction [1] , [14] . Much of the global conservation policy on endangered species is centered on land allocation schemes for securing source populations [15] , promoting and maintaining connectivity between fragmented populations [16] , [17] . Land is one of the most prized resources, and a major challenge to this conservation approach is the difficulty in convincing governments and policy makers on its allocation for conservation purposes. This problem is further compounded when objective criteria for delineating corridor habitats or documenting their functionality based on rigorous scientific data are lacking. As a result, conservation relies heavily on expert opinion and models of corridor connectivity that have little empirical validation.
The tiger ( Panthera tigris ) acts as a flagship species for the conservation of forested ecosystems throughout its range in Asia [18] . Conserving the tiger typifies the prospects and challenges inherent in the current paradigm of fragmented small populations and landscape based conservation models in large carnivores [19] . Extant tiger populations are confined to less than seven percent of their historical range in patchily distributed habitats across a range of twelve regional tiger conservation landscapes (TCLs) in southern and north-eastern Asia [20] . Six global priority TCLs of long-term tiger conservation significance are present in the Indian subcontinent. These Indian TCLs are important for global tiger recovery as they harbor over 50% of the estimated global population of ∼3,000 wild tigers [21] , [22] , and>60% of the global genetic variation in the species [23] . The high genetic variation seen in Indian tigers could be attributed to historically high population sizes, numbering about 50,000 individuals until c . 200 years ago, and habitat contiguity that permitted genetic exchange between the various regional tiger populations in the area [23] . Due to change in land ownership and forest use policy in the mid nineteenth century during British rule and again during the early years of India's independence a century later, much of the forest was cleared for timber and agricultural needs [24] , [25] . This change in land use combined with organized trophy hunting and bounty driven extermination resulted in severe decline, fragmentation and isolation of tiger populations throughout India [25] , [26] . Tiger conservation and subsequent population recovery in India began during the 1970s with the creation of a number of protected areas (Tiger Reserves) under the Project Tiger network in 1973 [18] , and aided by comprehensive wildlife legislation (Wildlife Protection Act 1972, [27] ). Under Project Tiger, the tiger was used as a flagship and umbrella species for conserving the biodiversity of India's forested ecosystems. However, even though extensive areas have been added to the protected area network, the future of tigers is under severe threat from commercial poaching, and extensive habitat fragmentation within the last two decades [15] , [18] , [21] . The rise in human-wildlife conflict and issues dealing with land rights of forest-based dwellers, as people are present both inside and outside tiger reserves, further vexes grass-roots conservation especially at a landscape-scale, and negatively impacts tiger dispersal capability and survival within TCLs [28] . These factors have precipitated the systematic decline in tiger and prey populations from both outside and inside reserves, as attested by the recent local extirpations in few areas [5] , [29] .
Currently in India, the once contiguous tiger population is now fragmented with source populations primarily restricted to tiger reserves. A tiger reserve is legally mandated to designate a critical core area wherein human habitation and resource extraction is not permitted (Wildlife Protection Act 1972, amendment 2005 [30] ). This core is surrounded by a buffer zone, which is essentially a multiple use area, wherein conservation objectives are to be given precedence over other land uses. Breeding populations of tigers are mostly located in the core area of tiger reserves, while the buffers usually serve as population sinks [22] , [28] , [31] . The size of these tiger reserves vary between 344 km 2 to 3,150 km 2 (average 1,321 km 2 ), with tiger densities ranging from about 0.1 to 20 individuals per 100 km 2 [22] , [31] , [32] . For a demographically viable tiger population, a minimum of 20 to 25 breeding units are believed to be essential [15] , [32] , [33] . As such, many extant tiger populations are by themselves inadequate for long-term persistence [33] , [34] , either because of habitats harboring a low number of breeding tigers, small size of the protected area and/or ecological isolation from other populations. High spatial genetic structuring and small population size observed in today's Indian tiger populations [35] dictates preserving them in a metapopulation framework wherein individual populations are connected through a permeable habitat matrix and can occasionally exchange individuals [36] , [37] . This would result in re-colonization of suitable habitat patches where tigers have become locally extinct and ‘rescue’ declining local populations from extinction by immigrants [37] , [38] . Understanding and managing the metapopulation framework of extant tiger populations is an important strategy for ensuring their long-term conservation. This approach entails strict preservation of source populations in protected areas and informed conservation strategies across tiger landscapes.
Due to the relatively high K selected life history traits of the tiger in comparison to other large cats, dispersal and immigration play a vital role in long-term viability of tiger populations [32] . Incidentally, it was likely due to the ‘rescue effect’ by immigrants from high-density populations and intact habitat corridors in the vicinity of Chitwan National Park, Nepal, which enabled the tiger population in the park during the 1930s to recover, even after heavy trophy hunting, to pre-decline levels in only three years [32] . In recent times, tigers have successfully recolonized Rajaji National Park, India, in the Shivalik-Gangetic Plain landscape, from connected source populations further east, within a decade of having completely disappeared from the area [39] . Small tiger populations that become isolated are likely to face extinction due to demographic stochasticity, inbreeding depression [40] and deterministic factors such as poaching [32] , [33] , as witnessed in the small and isolated Indian tiger reserves of Sariska and Panna which recently suffered from local extinction events, although tigers were later re-introduced [5] , [29] . Habitat connectivity is integral to sustaining regional populations of tigers, as they require contiguous forest connectivity for dispersal and genetic exchange between populations [41] . Currently, within the six tiger occupied landscapes of India, habitat contiguity varies extensively, with the best being within the Western Ghats and the North East, while fragmentation is highest in the Shivalik-Gangetic Plain and the Central Indian Landscapes [42] . Most of the connecting habitats in these landscapes are not within the legal domain of protected areas and are often lost to burgeoning development demands of a growing economy and attrition by human consumptive uses. In India, the transfer of forest-land to other land uses requires approval from the Federal Government as outlined in the Forest (Conservation) Act 1980 [43] . Since Federal Government approvals are usually sought on a case-by-case basis, and rarely are the cumulative impacts of projects or landscape scale conservation significance of forest patches factored into decision making, such permissions are frequently granted [44] . However, when the Supreme Court of India and Federal Government Committees were presented with concrete scientific evidence on the significance of conserving these forest patches, development projects even of national interests have been stalled [45] , [46] , [47] . Unfortunately, scientific data rarely exist to substantiate the landscape-level conservation significance of forest patches that constitute habitat corridors, and crucial areas are often lost. Studies on spatial dispersal and gene flow to detect population units and migration between patches can provide a quantitative and formal assessment of corridor function and identify priority populations for conservation action.
Assessing gene flow in species across populations in complex fragmented habitats is critical to understand how landscapes structure genetic variation and maintain metapopulation connectivity. Unfortunately, the traditional validation of habitat connectivity through the direct observation of individual animal movement is logistically difficult as it would entail following the fates of many radio-collared or camera trapped individuals over a regional scale and spanning multiple generations. As a result, alternative genetic assignment methods based on individual clustering approaches [48] , [49] have gained popularity [7] , [50] . The integration of metapopulation genetic models with spatial analytic tools in a landscape genetics framework provides a quantitative approach for understanding the role of geography, habitat and land-use features either as barriers or facilitators to gene flow among natural populations [51] . Though initially restricted to analyses correlating with linear distances [51] , [52] , the developing field of landscape genetics has now advanced to include more complicated connectivity modeling incorporating ways in which habitats are actually traversed in nature. The use of landscape heterogeneity patterns and habitat permeability obtained from Geographical Information Systems (GIS) layers to model habitat connectivity by least-cost pathway (LCP) analysis [53] and circuit theory based isolation-by-resistance (IBR) model [54] , [55] that permit gene-flow between populations provide an objective criteria for delineating and prioritizing habitat corridors. There is a small but rapidly growing body of literature investigating the relationship between genetic and corridor connectivity, with both LCP and IBR models finding promise in gene flow studies on taxa with lower dispersal capabilities and that readily form visible metapopulations such as amphibians (tiger salamanders, Ambystoma sp. [56] , [57] ), to wide-ranging carnivore species (cougar, Puma concolor [58] ; bobcat, Lynx rufus [59] ; wolverine, Gulo gulo [60] ; black bear, Ursus americanus [61] ). Where available, researchers have incorporated information from animal habitat use and movement behavior in the cost parameterization schemes to approximate realistic paths of least resistance, as in Reding et al. [59] . However, such data are not readily obtainable, and hence the vast majority of studies rely on expert opinion and a priori assumptions on animal presence to assign cost schemes and parameterize landscape resistance to gene flow. Although informative, the parameterization schemes used in landscape resistance surfaces to model movement paths and the assignment of cost schemes to grids in GIS rasters could easily introduce biases which may be more reflective of habitats as perceived by humans rather than by animals [62] , [63] . Incorporating information obtained from fine-scale species and landscape-specific ground data on suitable habitat, cover, prey availability, disturbance and threats in considering the attribute of surrounding cells, is one way which could help reduce subjectivity involved when assessing resistance or cost of a cell and the likelihood of path usage [63] .
In this study, we investigate patterns of landscape heterogeneity and spatial genetic structuring to identify barriers and minimal habitat corridors for gene flow between populations within the fragmented tiger habitats in Central India. The Central Indian landscape is a globally recognized area for tiger conservation, with significant potential for long-term persistence of the species [15] , [31] . The area supports one of the largest global concentrations of tiger populations (∼20% of an estimated 1,700 adult Indian tigers, [31] ) in patchily connected habitats. Although the populations were historically connected, rapid infrastructural development and urbanization in recent years threaten to form permanent barriers to dispersing tigers by isolating tenuously connected small populations, thereby effectively reducing long-term metapopulation persistence. Recent population and spatial genetic studies have observed low genetic structure among populations indicative of gene flow [64] , [65] and long-range dispersal which are affected by increasing urbanization in the area [66] . Although tigers can move huge distances in undisturbed habitats [67] , the complex fragmented habitat mosaic in the area, interspersed with high density human settlements and increasingly urbanized centers, have generally been thought to limit long-range dispersal [41] . Dispersal in tigers, like in lions ( Panthera leo [19] ) is male biased, as female offspring tend to reside and breed close to their maternal ranges, while male offspring disperse long distances and establish home ranges far from their natal areas [41] . This study explores a strategy that utilizes genetic assignment methods to detect population genetic structuring and determine which populations are in migratory contact, extensive occupancy modeling and GIS analysis to delineate structural connectivity between populations, and a correlation process between landscape connectivity versus population pairwise genetic distances to determine which of the movement cost schemes and modeled corridors best explain the observed genetic structuring in the area.
We extensively and intensively collected scat and a few tissue samples across seven tiger reserves in the Central Indian Landscape and first identify tiger individuals by genotyping the DNA extracts using eleven autosomal microsatellite loci. Next we assessed spatial genetic structuring and gene flow in the identified individuals through individual clustering methods. We use likelihood based [49] and Bayesian [48] , [68] assignment methods to detect first and second generation migrants between the identified genetic population clusters. Since resident tigers do not occur outside of forested habitat, we surveyed all of the forested area (76,913 km 2 ) within 185,100 km 2 of Central India. Based on our understanding of tiger ecology, we predicted a priori that tigers should occur in vast, undisturbed, productive forest patches, with high density of large wild ungulate prey, which would be negatively impacted by human disturbances [20] , [31] , [41] , [67] . We tested these a priori hypotheses by spatially explicit modeling of tiger occupancy that accounted for imperfect detections, using covariates obtained by remote sensing and ground surveys covering all forest patches within our study area. We then used this spatially explicit information of tiger occupancy as a resource selection probability function [69] , [70] to model habitat corridors joining tiger populations using LCP [71] and circuit theory [72] analyses in a GIS setting. With genetic data we tested if the observed population structure and dispersal between populations is in concordance with ground reality of tiger occupancy and existing habitat connectivity. Our comprehensive study highlights the importance of particular tiger source populations and intervening forest corridors for maintaining metapopulation structure within Central India. It provides a basis to formulate conservation policy and assist informed decision making for land-use planning at the landscape scale.
Materials and Methods
Ethics statement.
The majority of field sampling was conducted non-invasively from tiger scat, without animal capture and handling. Permits for collection of tiger scat samples were obtained from the National Tiger Conservation Authority and the State Forest Departments. Capture and radio collaring of tigers required the approval of the Ministry of Environment and Forests, Government of India and the Chief Wildlife Warden, Madhya Pradesh State, under the Wildlife (Protection) Act 1972. The permissions define the conditions required for capture of tigers, which include an approved protocol and participation by a Park Official and supervision by a qualified veterinarian in the capture and collaring exercise. Both these permissions were obtained and strictly adhered to. Capture operations were conducted by trained veterinarians and wildlife biologists as per the protocols of the Wildlife Institute of India and the National Tiger Conservation Authority. A tiger tissue sample was obtained from Satpura Tiger Reserve where the tiger died due to natural causes (was killed by another tiger in a territorial strife). This research project was conceived and radio collaring reported in this paper was done prior to the formation of an animal ethics committee at the Wildlife Institute of India.
The present study was carried out in the global priority tiger conservation landscape of Central India within the states of Madhya Pradesh, Maharashtra, and Chhattisgarh ( Figure 1 ). A forested area of 76,913 km 2 (20.1–23.5°N and 76.5–81.5°E) covering the seven tiger reserves of Melghat, Satpura, Pench, Kanha, Tadoba, Achanakmar, and Bandhavgarh along with their buffer zones, corridor habitats and adjoining forested habitats were sampled. Sampled sites covered different types of tiger habitats found in Central India ranging from the tropical moist-deciduous Sal ( Shorea robusta ) forests in Kanha and Bandhavgarh to tropical dry-deciduous teak ( Tectona grandis ) dominated forests in Pench, Tadoba and Melghat. The topography varied from about 200 meters above sea level (m a.s.l.) in the low-lying hills to the dadar plateaus and meadows in Kanha (500 m a.s.l.) and the rugged Satpura ranges (highest elevation 1,352 m a.s.l.). The rainfall, primarily restricted to the monsoon season (late June to September end) ranged between 1,000 to 2,200 mm per year. The large mammal fauna found in the region included tigers ( Panthera tigris ), leopards ( P. pardus ), sloth bears ( Melursus ursinus ), dholes ( Cuon alpinus ), gaurs ( Bos gaurus ), wild pigs ( Sus scrofa ), sambar deer ( Rusa unicolor ), chital deer ( Axis axis ), barking deer ( Muntiacus muntjak ), swamp deer ( Rucervus duvaucelii ) and nilgai antelopes ( Boselaphus tragocamelus ).
- PPT PowerPoint slide
- PNG larger image
- TIFF original image
The study area of Central India spanning the states of Madhya Pradesh, Maharashtra and Chhattisgarh, showing tiger habitat (forest cover) coded with tiger occupancy probability, protected areas, human habitation (night lights), major roads and least-cost habitat corridors connecting tiger reserves. Individually genotyped tigers (n = 165) are shown as color coded dots at their sampled locations with their colors matching their genetically assigned population.
https://doi.org/10.1371/journal.pone.0111207.g001
Genetic Sampling and Laboratory Work
Blood from 16 radio-collared tigers, one tissue sample from a dead individual and 587 putative tiger scat (faeces) samples were collected between 2006 and 2011 from the seven Tiger Reserves, and at a few intervening forest corridors in the area ( Figure 1 and Table 1 ). The number of samples obtained was largely proportional to the population size of tigers in that region. However, due to logistical constraints the Tadoba population was under sampled. Scats were stored, either dry with silica or in 75% ethanol, and kept at ambient temperature, prior to laboratory analysis. For each scat, a Global Positioning System (GPS) reading was taken and transferred into a GIS. Scat DNA extractions were performed in a room dedicated to low-copy DNA extraction, using the guanidine isothiocyanate - silica extraction protocol [73] . For every extraction, negative controls composed of reagent only without the scat sample were included to monitor contamination. Extractions from blood and tissue samples were carried out using the DNeasy blood and tissue kit (QIAGEN, Germany).
https://doi.org/10.1371/journal.pone.0111207.t001
Tiger scats were identified from among the field collected scats through PCR and Bam HI restriction enzyme digestion of the mitochondrial DNA cytochrome b gene ( Figure S1 ; see Methods S1 and Tables S10 and S11 for details on protocols and reference species used for PCR). Unambiguously assigned tiger scats and blood and tissue samples were individually identified using a panel of eleven microsatellite loci, derived from domestic cat [74] and tiger [75] , [76] . The loci consisted of three dinucleotide (Fca304, Fca954, 6Hdz700), three trinucleotide (Pati01, Pati09, Pati15) and five tetranucleotide repeat markers (Fca441, F85, F53, F124,Pati18), variously labeled at the 5′ end of each forward primer with 6–FAM, PET, VIC and NED dyes. Gender identification in individual tigers was carried out by amplifying the Y chromosome linked SRY gene and an X chromosome microsatellite locus Fca651. PCR amplifications were carried out in 10 µl reactions with a multiple panel of 3 to 4 loci using the Multiplex PCR kit (QIAGEN) according to the manufacturer's instructions. Amplified products were resolved on the ABI 3130 Genetic Analyzer and GENEMAPPER 3.7 (Applied Biosystems, USA) was used to score allele sizes. To limit genotyping error due to allelic dropout (nonamplification of one allele in a heterozygote), multiple PCR replicates were conducted as in Navidi et al. [77] . Heterozygotes were confirmed with at least two independent replicates and homozygotes with five replicates. The genotype data were checked on MICROCHECKER [78] for identifying and correcting genotyping errors such as those that arose from stuttering patterns, null alleles and small-allele dominance.
Identification of Individuals and Descriptive Statistics
In order to investigate the power of the eleven microsatellite loci to distinguish among closely related individuals in the same population, the conservative sibling probability of identity ( PI sib ) statistic [79] , [80] was computed in GIMLET 1.1 [81] . Unique multilocus genotypes were identified using the Identity analysis option in CERVUS 3.0 [82] , [83] . Samples that showed mismatches at up to two loci were re-examined for possible genotyping errors and allelic drop-out, and again amplified thrice in order to confirm the multilocus genotypes before assigning them as unique individuals. Multiple replicates of the same individual from the same locality were discarded and only unique multilocus genotypes were used for all further analyses. We used CERVUS 3.0, GENEPOP 4.1 [84] , [85] , GENALEX 6.3 [86] and FSTAT 2.9.3 [87] to calculate the following descriptive statistics: (i) number of alleles per locus, (ii) observed and expected heterozygosity, (iii) tests for deviation from Hardy-Weinberg Equilibrium (HWE), (iv) significance values for linkage disequilibrium (LD) among all pairs of loci, and (v) estimates of population pair-wise F ST [88] and R ST [89] values. Loci with null alleles were flagged by MICROCHECKER, and we tested for deviation from HWE using both null allele adjusted and unadjusted (observed allele frequencies) genotypes. We also tested for associations between genetic variation and estimated population size through Spearman's rank correlation using the pspearman package [90] in R ( http://cran.r-project.org ).
Population Genetic Structure
We used two types of individual-based analyses to assess genetic differences among individuals and assignment patterns of tigers to populations. First, a Principal Coordinates analysis (PCoA) based on pair-wise Phi PT genetic distances [91] was carried out in GENALEX and the scatter of population-wise individual assignments was plotted on the first three PCo axes using NCSS ( www.ncss.com ) to understand broad spatial patterns of populations structure in the landscape. Next, we used the Bayesian individual clustering approach in STRUCTURE 2.3.3 [48] to detect population structure among sampled localities in the area by assigning sampled individuals into a number of clusters ( K ) based on the multilocus genotype data alone. The clustering process ensures that for identified population clusters, deviations from Hardy Weinberg and linkage equilibrium are minimized. We analyzed our data in STRUCTURE by using the admixed model and correlated allele frequencies option to carry out thirty independent simulations at each K ( K = 1 to 10), with a burn-in length of one million Monte Carlo Markov Chain (MCMC) steps and data collection phase of five million MCMC iterations. These run times were sufficient to ensure convergence of the Markov chains, and all runs were carried out both with (locprior = 1) and without (locprior = 0) using prior sampling locality information. The true K or most likely number of population clusters in the dataset was inferred from (i) the ad hoc parameter of log-likelihood change in probability of individual assignments to K clusters ( Ln P(K) , [48] ), and (ii) the second order rate of change in the likelihood of K values ( delta K , [92] ). Both these values were computed from the STRUCTURE output using the program STRUCTURE HARVESTER v0.6.91 [93] . We also carried out an Analysis of Molecular Variance (AMOVA, [91] ) in GENALEX to compare the population clusters identified by the STRUCTURE analysis. Genetic variances were partitioned at two levels, viz. among all the STRUCTURE identified population groups ( K clusters) and among subpopulations within each group.
Detection of Migrants
We used three Bayesian approaches implemented in STRUCTURE 2.3.3 [48] , GENECLASS 2.0 [49] and BAYESASS 1.3 [68] , for identification of migrant and admixed individuals. STRUCTURE was used to calculate the posterior probability of whether individuals are residents of their sampled population or migrants from other areas by incorporating the previously identified population cluster information with a priori designation of the migration rate (MIGPRIOR = 0.05). All other parameters and run times followed previous population clustering runs (as detailed above in the preceding methods section on analyzing population genetic structure). We detected no biases in a priori assignment of the migration rate as the selection of particular MIGPRIOR values (0.001 to 0.1) did not substantially influence the STRUCTURE output, therefore only results for MIGPRIOR = 0.05 are presented here.
Next, we used the likelihood-based estimator L h /L max in combination with the resampling algorithm of Paetkau et al. [94] , implemented in the ‘detect migrants' function in GENECLASS to exclusively identify first generation migrants, i.e. individuals assigned to a different population other than the sampled population. The Paetkau et al. [94] routine was selected on basis of its superior simulation scheme which closely mimics natural processes and results in accurate type I error rates. L h /L max , is the ratio of L h , the likelihood of a given individual being assigned to its sampled population to L max , the greatest likelihood among all sampled populations [94] . We employed the Bayesian criterion of Rannala and Mountain [95] in combination with the re-sampling algorithm of Paetkau et al. [94] using a simulated set of 10,000 area-specific genotypes, to determine migrant thresholds (type I error α levels of 0.01 and 0.05) of L h /L max . We considered a minimum log likelihood L h /L max ratio of 2.0, which corresponds to a 100 times probability of being cross-assigned, as the threshold level for determining putative migrant status of an individual [7] .
Third, the non-equilibrium Bayesian assignment test of BAYESASS was used to trace each individual's immigrant ancestry within the last two to three generations. Unlike STRUCTURE and GENECLASS analyses which require loci to follow Hardy-Weinberg and linkage equilibrium, BAYESASS is robust to violations from Hardy-Weinberg equilibrium as it measures contemporary gene flow within the last few generations based only on multilocus allele sharing among individuals [68] . To ensure convergence, a total run length of eight million MCMC iterations was performed, of which the first two million runs were the burn-in phase and the remaining six million runs comprised the data collection phase with the MCMC chain being sampled every 2,000 steps. Individual assignments and immigrant ancestries were calculated at a migration rate prior of 0.05, and keeping all other settings at default. Varying the prior rate (0.01 to 0.15) did not affect the results.
Lastly, to corroborate the results of the above assignment based migrant decisions, a likelihood based parentage analysis was carried out in CERVUS 3.0 [82] , [96] to identify likely parent-offspring relationships between putative migrants and an individual in the cross-assigned source population based on log of the odds (LOD) scores. The LOD score that is the natural logarithm of the overall likelihood ratio for each candidate parent is calculated by multiplying together the likelihood ratios at each locus. A positive LOD score means that the candidate parent is likely to be the true parent, whereas a negative value means that the candidate parent is less likely to be the true parent. We estimated LOD scores for strict (95%) and relaxed (80%) confidence limits as 7.0 and 4.9 respectively, which were calculated from a simulated set of 10,000 offspring and 300 candidate parent genotypes, assuming 25% of candidate parents were sampled, 93% of loci were typed with typing error of 0.01 to 0.10.
Designation of migrant status to an individual was contingent upon - (i) significant assignment of first generation migrant status in GENECLASS ( P <0.01, Lh/Lmax ≥ 2.0); (ii) observance of>50% migrant or cross-assignment probability in STRUCTURE and BAYESASS; (iii) high assignment probability to first generation immigrant ancestry state (gen1>50%) in both STRUCTURE and BAYESASS; and (iv) high membership ( Q >0.8) to a single non-home cluster in the STRUCTURE analysis without prior population information. Further, in most cases of putative migrants, the successful parentage assignment corroborated the migrant status of the individual tiger. We considered a conservative approach by identifying individuals as putative migrants only if all three programs suggested evidence of immigrant ancestry.
Estimation of Contemporary and Historical Migration Rates
In order to study gene flow across different timescales, we used the programs BAYESASS 1.3 [68] and MIGRATE 3.3.2 [97] , [98] to compare migration rates over contemporary and historical timescales, respectively. Although the two programs use different approaches to derive estimates of gene flow, both programs generate parameters from which a comparative estimate of the proportion of genetic migrants in the population per generation ( m ) can be inferred. In BAYESASS, a Bayesian approach incorporating an MCMC sampling scheme is used to estimate migration rates between pairs of populations over the last few (approximately <5) generations back. With an estimated generation time of four to five years in tigers [99] , this period corresponds to a timescale of nearly 20–25 years ago. MIGRATE on the other hand, uses the coalescent to estimate the relative mutation-scaled effective population size, theta, θ Ne (4 N e µ ; where N e is the effective population size and µ is the mutation rate) and asymmetric mutation-scaled immigration rate M ( m/µ ). The mutation-scaled immigration rate M , which is the immigration rate m divided by the mutation rate µ , is a measure of the importance of immigration events over mutation in contributing to variation in the population [97] . The relative effective population size, theta, is the number of individuals representing an idealized (Wright-Fisher) population that will result in the same amount of genetic drift as in the actual population. The number of migrants per generation, 4 N e m , is the product of theta and M . MIGRATE assumes mutation-migration-drift equilibrium with values of M and theta constant over time and parameter estimates in MIGRATE date back nearly 4 Ne generations into the past (approximately thousands of years ago). Hence, these migration rates provide estimates of gene flow that post and pre-date the estimated time (approximately 600 years ago) when humans began to significantly alter the habitats in which these tigers currently live.
For both the BAYESASS and MIGRATE runs, we used the STRUCTURE defined population clusters to estimate pairwise migration rates. BAYESASS runs were performed as described in the preceding methods section on detecting migrants. A total of 8×10 6 MCMC iterations were carried out, by discarding the first 2×10 6 steps as burn-in and sampling at every 2,000 iteration intervals of the remaining 6×10 6 MCMC chain. Runs were carried out with a migration rate prior of 0.05 while other parameters were kept at default settings. The average result from three independent BAYESASS runs is presented. Estimates of historical gene flow and effective population size were carried out in MIGRATE by using the Bayesian approach and the Brownian motion model as an approximation for the step-wise microsatellite mutation model. Following initial trial runs, the Bayesian search criteria for the MCMC sampler was set at 10 replicates of one long chain of 50,000 steps with every 100 steps of the chain being recorded, producing a total of 5×10 7 visited parameter values. The initial 10 7 steps of the MCMC run were discarded as burn-in, and the remaining 4×10 7 runs were sampled. To increase efficiency of the sampler, we used four chain-heating temperatures of 1, 1.5, 3 and 10,000, which allows a more efficient exploration of the genealogy space. We used parameter estimates from the initial run to calculate starting values of theta, for use as new parameters during subsequent runs. Parameter estimates from the final run were similar to the results of the initial runs. All MIGRATE runs were carried out on the Bioportal cluster computing facility at the University of Oslo, Norway ( https://www.bioportal.uio.no/ ; accessed 12 May 2013).
Detection of Genetic Bottleneck
To detect past occurrences of genetic bottleneck in the sampled populations, we evaluated three summary statistics - (i) Wilcoxon's sign rank test and (ii) mode-shift test, implemented in the program BOTTLENECK 1.2.02 [100] , and (iii) M ratio test [101] implemented in ARLEQUIN 3.1 [102] . We were not interested in quantifying population expansion/decline or dating the time of and therefore avoided using other considerably lengthy Bayesian procedures [103] , [104] . Wilcoxon's test detects bottlenecks based on the probability of heterozygosity excess over that expected at mutation-drift equilibrium in a population. It is most effective at detecting historic bottlenecks occurring over approximately 2–4 N e generations in the past. The mode-shift test is more suited for detecting bottlenecks within the last few dozen generations [105] , [106] . This test is based on the premise that a stable population at mutation-drift equilibrium will have a large proportion of alleles at low frequency and a smaller proportion at intermediate frequencies and few at high frequencies. The resulting allele proportions yield an L-shaped distribution. In bottlenecked populations the mode is shifted because of the rapid loss of alleles present at low frequency. We ran 10,000 simulations using the program BOTTLENECK in 5 populations (excluding KPC, ATR and TATR) under both the stepwise mutation model (SMM) and the two-phase mutation model (TPM) with 95% single step mutations and 5% multi-step mutations and a variance of 12 as recommended by Piry et al. [100] . P -values from the Wilcoxon's test were used to examine bottlenecks at each timescale and were assessed at the alpha 0.05 level. The M ratio ( M = k / r +1) was calculated from the mean ratio of the number of alleles ( k ) and the allele size range ( r ). Assuming loci follow a generalized stepwise mutation model, the loss of rare alleles would diminish the value of k at a faster rate than r thereby a drop in the M ratio below a threshold of 0.68 would be suggestive of populations that experienced a recent bottleneck [101] .
Field Data Collection for Occupancy Analysis
(a) tiger sign surveys..
The entire study area was divided into 10×10 km grids. Each grid that contained potential tiger habitat (all types of forest cover) was surveyed by replicate search paths for tiger sign. The number of surveys per grid ranged from 3 to 35, and was proportional to the amount of tiger habitat within each grid. Areas under agriculture, industry, and human habitation that were known to be non-habitat for tigers were not surveyed in an occupancy framework. Each survey consisted of a 5 km search for tiger signs with approximately one survey for every five km 2 of habitat. Surveys were not random, but instead conducted along features that were likely to have tiger sign (e.g. dirt roads, dry water courses, and animal trails) so as to maximize detections [22] . Surveys were conducted by the local guard and a local assistant who had intimate knowledge of the forest and were trained to observe and record tiger sign in pre-designed datasheets. All encounters of tiger pugmark track sets and scats were recorded. These were distinguished from those of other carnivores based on criteria described by Jhala et al. [107] and Karanth and Nichols [108] . A total of 79,000 km of search effort was invested in 15,800 replicate surveys between December 2009-February 2010 (cold and dry season) across the entire study landscape to adhere with the assumption of occupancy closure [70] and have minimal influence of weather (rainfall) on sign detections and distribution. A total of 1,851 grids were sampled.
(B) Prey Assessment.
Within each forest beat, one or two permanent line transects of two to four km in length were delineated. Each transect was walked on two or three subsequent mornings (06:30 to 08:30 hrs) by two observers to record encounter rates of wild ungulates and domestic livestock. Data on number of each species seen and the length of transect were recorded to compute encounter rates of each species. In disturbed forests (outside of protected areas) wild ungulate densities were low, animals were shy, and difficult to record using line transects. Therefore, at every 400 m along the line transect we also sampled a plot of 20×2 m to record ungulate dung. Dung was visually distinguished to species [107] and dung density for each species, wild ungulates as a group, and domestic livestock was computed separately. Encounter rates of ungulates and dung density were used as indices of ungulate abundance. The number of transects within each 100 km 2 grid ranged from 1 to 24, and were proportional to the quantum of tiger habitat within that grid. The total effort invested in transect surveys was 71,468 km of walking on 26,688 occasions.
(C) Human Disturbance.
At every 400 m along transects established for ungulate assessment a plot of 15 m radius was sampled to assess indices of human impact. Presence of (a) human/livestock trails within the plot, and (b) sighting of humans and livestock from the plot were recorded [107] . The number of plots within a 100 km 2 grid ranged from 5 to 120. The total number of plots sampled across the landscape was 51,073.
Remotely Sensed Variables
Remotely sensed data that depict landscape characteristics and human impacts were obtained from various sources and extracted at the 10×10 km grid resolution. Forest cover was obtained from the Forest Survey of India [109] that is based on IRS 1D LISS III satellite with 4 multispectral band data at 23.5 m resolution. Normalized Differential Vegetation Index (NDVI) information were derived from 1 km 2 Advanced Very High Resolution Radiometer (AVHRR) data, acquired from the National Aeronautics and Space Administration's (NASA) Television Infrared Observation Satellite (TIROS) ( http://science.nasa.gov/missions/tiros/ ; accessed 23 Dec 2010). Road and drainage information were obtained from Digital Chart of the World ( http://statisk.umb.no/ikf/gis/dcw/ ; accessed 20 Dec 2010). Protected area shape files were obtained from the wildlife database at the Wildlife Institute of India, National Tiger Conservation Authority and State Forest Departments of India. The Shuttle Radar Topography Mission has produced the most complete, high-resolution digital elevation model of the earth [110] . Within each 1 km 2 grid, this information was used for computing average elevation and the coefficient of variation of elevation used as a measure of terrain ruggedness. Night light data was obtained from the United States Air Force Defense Meteorological Satellite Program (DMSP) and National Oceanic and Atmospheric Administration's (NOAA) Operational Linescan System (OLS) ( http://www.ngdc.noaa.gov/dmsp/sensors/ols.html ; accessed 18 Dec 2010). Density of roads (length of paved road per km 2 ), and Euclidean distances to roads, protected areas and night lights were computed in ArcGIS 9.3 ( www.esri.com ) software.
Occupancy Modeling
Tiger site occupancy was a priori expected to be positively influenced by (a) prey abundance, and (b) amount and quality of tiger habitat, and negatively influenced by (c) human disturbance [22] , [115] , [116] . We tested these hypotheses by modeling variables representing these factors as covariates using the logit link function in PRESENCE [70] , [114] . We initially generated data on 23 site covariates that represented landscape and habitat features (forest area, core forest area, forest patch size, NDVI, elevation, ruggedness, drainage density, rainfall, distance to protected area), prey availability (chital, sambar, wild pig and gaur encounter rates on line transect walks, and wild ungulate dung density), human disturbance (distance to night lights, distance to roads, humans and livestock encountered on transect walks, human/livestock trails within sampled plots, and livestock dung density) that could potentially explain tiger occupancy. These covariates were examined with exploratory data analyses for their interrelationships and relationship to tiger presence (by scatter plots, box plots, and correlation analysis) and based on this a subset of 16 variables was selected for inclusion as site covariates for occupancy modeling (see Table S1 for univariate coefficients). Since many of the covariates had high correlation coefficients between them, their contribution to explaining tiger occupancy would be redundant. To account for this collinearity and to reduce the dimensionality of the covariate matrix we extracted Principal Components (PCs) from 16 variables [117] . The varimax rotated PCs were further used as independent variables in a logit link function to model tiger occupancy in the program PRESENCE ( [70] , available for download from http://www.proteus.co.nz/ ). Model selection was done using AIC, and model fit was assessed by comparing the actual detection histories with simulations generated from 50,000 parametric bootstrap runs of the target model in PRESENCE. The over dispersion parameter Ĉ close to one suggests that the model provides an adequate description of the data, values of Ĉ greater than one suggests more variation in the data than expected by the model, and values less than one suggest less variation in the data. The standard errors of model estimates were adjusted by the square root of Ĉ as recommended by MacKenzie et al. [70] . Models were built using PCs that represented prey abundance, human disturbance and habitat quality; these were evaluated against the null model and the full model by their delta AIC values. A total of six models were evaluated for modeling tiger occupancy and coefficient estimates for all models with delta AIC <4 were averaged based on model weights [70] to arrive at occupancy probability ( Ψ ) in each grid.
Tiger Population Extents and Occupied Habitats
We used two approaches to estimate population extents and area of occupied habitats; (i) a more conservative approach wherein we considered only those grids that detected tiger sign as being occupied (the naïve estimate) and (ii) model inferred occupancy that corrected for detection bias and covariates in PRESENCE. Herein, landscape scale occupancy was computed by sum of cell occupancy probability values and divided by the total number of cells. Tiger habitat (forested area) in each grid was weighted by the tiger occupancy probability of that grid and summed across all grids to arrive at occupied tiger habitat for the landscape [115] . All adjacent tiger sign detected cells were joined and were considered to be occupied by a single tiger population.
Habitat Corridor Modeling
Grid based tiger occupancy probability ( Ψ ) obtained from PRESENCE was used as a measure of habitat suitability for tigers [69] , [70] . A cost surface for tiger habitat suitability across grids was generated as 1- Ψ . For the non-tiger habitat (human land uses) where tiger occupancy values were not available, we considered them permeable to tigers at higher costs than forested habitats, although human habitations (townships) were considered impermeable to tigers (see Methods S2 for details). These costs were used as a resistance layer for modeling habitat connectivity using LCP [63] and circuit theory [118] analyses. Based on these cost surfaces the resulting models would optimize connectivity by selecting high quality tiger habitat and minimize gaps formed by non-tiger habitat. Least-cost pathways (LCP) were modeled using PATHMATRIX [71] , and resistance pathways were modeled using CIRCUITSCAPE [72] . Core areas of tiger reserves were considered as “sources” or areas of high potential from which tiger movement across paths of least resistance were modeled across the landscape. PATHMATRIX models several potential routes in a radiating manner from the “source” to connect to another adjacent “source”. It then selects a single “least cost” pathway as the best alternative. CIRCUITSCAPE models connectivity through habitat swaths considering resistance to movement based on pixel cost and corridor width [118] . It provides one to several potential alternatives for joining sources and helps in identifying bottlenecks within the corridors. Since the Central Indian landscape is highly fragmented and human dominated with clearly defined and limited forested habitat, we could overlay LCP on high resolution Google Earth images and align them to match geographic features within occupancy grids, to delineate realistic corridors. These least cost corridors buffered by 1.5 km (LCC) were considered the minimal essential corridors joining two tiger reserves.
Genetic Structure, Migrants and Corridor Cost
The genetic structure between populations is a consequence of the amount of genetic drift to which each local population has been subjected, due to its local effective size, and/or due to its degree of demographic, geographic and ecological isolation [119] . Since all the Central Indian tigers likely belonged to a large, mostly contiguous population till a few hundred years ago [23] , pairwise genetic distances between populations should reflect levels of differentiation and barriers to gene flow, i.e., the cost of movement between these populations. The proportion of migrants between population pairs would reflect gene flow in current or recent times while F ST values would indicate genetic differences over historical, more long-term time scales. We therefore expected to have more migrants detected between geographically closer populations that had lower movement costs between them. This exploratory analysis would corroborate the short-term mechanisms (migration events) that result in long-term ( F ST ) genetic differences between populations due to tiger movement across the landscape.
Pairwise F ST , R ST and Phi PT genetic distance estimates, obtained from AMOVA analysis in GENALEX, were linearized using the formula F ST /(1 - F ST ), as given by Rousset [120] . In order to determine which spatial model best explained genetic structuring, matrices of linearized pairwise genetic distances were correlated against matrices representing geographic distances (GGD), log-transformed geographic distances (log 10 GGD), least-cost pathway distances (LCPD) least-cost corridor distances (LCCD), and resistance distances (RD). In addition, the effectiveness of the modeled corridors and spatial distance matrices was compared using partial correlations that allowed one model to be tested, while controlling for other competing models [55] . The biologically realistic model not only exhibited the highest significant, positive correlation, but also displayed significant positive partial correlations after controlling for each of the competing models. Mantel [121] and partial Mantel [122] tests were carried out with 10,000 randomizations in the program zt [123] to evaluate the significance of the correlations.
As patterns of isolation-by-distance (IBD) are known to bias tests of hierarchical structuring and vice-versa, we used the population clusters as a covariate in a partial Mantel test, to model the partial correlations between pairwise genetic distances and spatial distances, while controlling the effect of population clusters (following Meirmans [124] ). Partial correlations between matrices representing pairwise genetic distances and spatial distance matrices were calculated with a third matrix describing whether population comparisons were made between (1) or within (0) the STRUCTURE identified clusters. A non-significant or negative partial correlation of genetic with geographic distance, after controlling for population clusters, would rule out patterns of underlying IBD in the observed genetic structure.
Identified Individuals and Descriptive Statistics
Out of 587 scat samples, 330 scats were successfully PCR amplified using the felid specific mitochondrial DNA cytochrome b (mtDNA cyt b ) primers, of which 275 were identified as tiger scat based on Bam HI restriction enzyme digestion profile of the PCR products ( Table 1 and Figure S1 ). From the 275 tiger scats, only 250 samples yielded microsatellite genotype data at a minimum of seven loci to be considered for Identity analysis in CERVUS. We identified 169 individuals with 81 recaptures from a total of 267 microsatellite loci genotypes (250 scats, 16 blood and 1 tissue). The total number of individuals identified here constitutes roughly 49% of the estimated total tiger population in the entire sampled area ( Table 1 ). Sex identification yielded 74 males and 85 females, with the sex ratio being nearly symmetric in most localities, except in the lower sampled areas. On average, individual multilocus genotypes were 93.2% complete. We included 97% (164 out of 169) of samples, those that had complete or near complete genotypes with a maximum of two missing loci, for further analyses. Two Tadoba individuals with three missing loci (73% complete) and three individuals from the Kanha Pench corridor with four missing loci (64% complete) were also retained since sample size from these areas was small and the genotypes represented unique tigers. The panel of eleven microsatellite loci that was used for individual discrimination had very low cumulative sibling probability of identity ( PI - sib ) of 1.5×10 −4 ( Table S1 ), indicating very high power to discriminate individuals. Even in the samples with the least amount of genotype information (four missing loci), the cumulative PI-sib value (1.6×10 −3 ) of the samples was sufficiently low to determine unique individuals.
No significant evidence of linkage disequilibrium (LD) among all pair-wise loci combinations was observed, when all sampling locations were pooled ( p >0.05 at 1,000 permutations). Except for deviation at three loci, Fca441, Pati09 and Pati18 ( p <0.001), all other loci were in Hardy-Weinberg equilibrium (HWE) in the pooled population after adjusting the critical P -value using the Bonferroni correction procedure ( Table S1 ). Loci not in HWE or with null allele frequencies>5% appear to be random with respect to population ( Table S2 ). Pati09 showed significant deviation from HWE across three populations, while Pati01 and Fca441 showed significant deviation from HWE in two populations. 6Hdz700, F53 and Fca304 each deviated from HWE in one population. Such departures from HWE could indicate the possibility of genetic structuring among populations and likely presence of related individuals in the data. The deviation from HWE in few populations could also be explained by the presence of null alleles in the data. While MICROCHECKER tests did not show any evidence of scoring errors due to stuttering or small allele dominance in the dataset, five loci (Pati01, Pati15, Pati18, Fca304 and F53) with high null allele frequencies>9% were detected ( Tables S1 and S2 ). Three loci deviating from HWE were likely due to the presence of null alleles in the data - Fca304 in Bandhavgarh, and Pati01 and F53 in the Melghat-Satpura-Tadoba cluster, as they showed significant deviation from HWE only with observed allele frequencies and not with null allele corrected frequencies (not shown). Although Pati15 and Pati18 also contained null alleles at high frequencies (>9%) in Kanha, they did not deviate from HWE in any population. Since these patterns were random with respect to population (e.g., no departures from HWE in Kanha, and null allele frequency <5% in Pench), it was more likely that these deviations could be due to the presence of population structuring in the data. Hence we retained all eleven loci in subsequent analyses.
Most tigers genotyped in this study showed high heterozygosity ( Figure S2 ). The minimum number of heterozygotes observed across loci for an individual tiger's multilocus genotype was two and three heterozygous loci, for one and two tigers respectively. The most polymorphic individuals (n = 5) were heterozygous at all eleven loci. Individuals that were completely homozygous at all eleven loci, were not observed. Nearly 90% of the tigers (i.e. 152 out of 169 individuals) had heterozygous genotypes at five to nine loci, and 12 tigers were heterozygous at ten to eleven loci. Genetic diversity estimates showed a mean number of alleles per locus to be 9.1±2.2 with heterozygosity He to be 75.4%±3.9 and Ho to be 70.1%±5.9 in the pooled population ( Table 2 ). A summary of population-wise genetic variation, revealed significantly higher allelic diversity and heterozygosity levels in the larger populations of Pench and Kanha compared to all other localities ( Table 2 and Table S2 ). The number of population diagnostic private alleles was high in Pench (n = 8) and Kanha (n = 6), while individual frequency for a private allele was highest in Bandhavgarh compared to all other localities ( Table S3 ). Estimated population size was positively and linearly correlated to genetic diversity statistics (Number of alleles, rho = 0.857, p = 0.011; polymorphism information content, rho = 0.738, p = 0.0458; Shannon's index of diversity, r = 0.714, p = 0.058). Partial correlations between estimated population size and the allele diversity indices, after controlling for the effect of sample size, were positive and highly significant (Number of alleles, r = 0.82, p = 0.012; polymorphism information content, r = 0.659, p = 0.054; Shannon's index of diversity, r = 0.714, p = 0.036). Heterozygosity values were not correlated with population size ( Ho : rho = −262, p = 0.428; He : rho = 0.357, p = 0.389). Correlation of estimated population size with actual sampled size was highly significant ( r = 0.964, p = 0.001), meaning that our samples were in proportion to the size of the population.
https://doi.org/10.1371/journal.pone.0111207.t002
According to the results of the Principal Coordinates Analysis (PCoA) based on Phi PT genetic distance estimator, individuals in the area were clustered into roughly four groups with varying degrees of population partitioning ( Figure 2A ). The three coordinate axes accounted for 60% of the variation in the dataset. Tigers were observed to cluster in four major groups. Tigers from Kanha, Pench and Melghat formed three distinct clusters that partially overlapped each other, while Bandhavgarh tigers formed a discrete cluster with minimal overlap. Tigers from Satpura, Tadoba, and Achanakmar were scattered within the clusters formed by Kanha-Pench-Melghat.
( A ) Three dimensional plot showing partitioning between different populations as obtained from PCo analysis based on Phi PT co-dominant genetic distance among individuals. ( B ) Summary barplot of STRUCTURE run at K = 4 showing population assignments for each individual. Four distinct population clusters are observed. Sampled populations are Melghat (M), Satpura (S), Pench (P), Kanha-Pench corridor (KPC), Kanha (K), Achanakmar (A), Tadoba (T) and Bandhavgarh (B).
https://doi.org/10.1371/journal.pone.0111207.g002
Calculation of delta K from the output of the STRUCTURE runs using prior population information (locprior = 1), produced the largest modal value of the statistic at K = 4, suggesting pronounced population subdivision at K = 4 ( Figure S3 ). On the other hand, the log-likelihood L(K) value reached an inflection point at K = 4 before gradually plateauing at K = 6 to 7 and finally leveling off at K = 8 ( Figure S3 ). The variance in L(K) increased at higher values of K , as reported previously with other studies [92] , [125] . The disparity in population structuring patterns between delta K and log-likelihood values occasionally occurs in cases where the F ST values are significant [126] . Examination of individual Q summary barplots ( Figure S3 ) yielded identical clustering patterns at all runs between assumed K = 2 to 4 (carried out both with and without prior sampling location information), and distinct population saturation, indicative of population subdivision was evident at K = 4 in conformance with the delta K approach. Based on the above, the four cluster solution ( K = 4) best describes the levels of genetic subdivision in our sample of the Central Indian tiger population ( Figure 2B ).
The four population clusters identified by STRUCTURE were similar to the clusters observed using PCoA plots, and in consonance with geographic configuration as well ( Figure 1 ). Melghat, Satpura and Tadoba represented populations with patchy connectivity and formed a unique cluster in the western and southern limits of the landscape, with few individuals being cross-assigned to the Pench and Kanha clusters. To the east of Melghat and Satpura, Pench formed a unique cluster. A few individuals in Pench had cross-assignments with Kanha suggesting gene flow between these two population clusters substantiated by a functional habitat corridor between Pench and Kanha. The next cluster in the eastern part of the landscape was represented by tiger populations in Kanha and Achanakmar. Individuals from the forested corridor between Pench and Kanha were also assigned to this cluster, but most had mixed assignments to both populations indicating that this was not a distinct but rather an admixed population. The last cluster was represented by Bandhavgarh, in the north-eastern part of the landscape, which formed a distinct isolated population where all individuals were assigned to the sampled locality.
Partitioning of genetic variation in the AMOVA test indicated low but significant differentiation ( p <0.01) between the STRUCTURE identified clusters ( Table S4 ). The major portion of genetic variance was found within populations (88%) with 7% among the population clusters and 5% among populations within clusters. Exact tests showed significant genetic variance on all three levels ( p <0.01). Both F ST and Phi PT values showed highly significant structuring ( p ≤0.001), and had relatively similar trends in magnitude with low sampling variance. In contrast, R ST estimates showed no variation between groups, and had unreliably high sampling variances and mean square error estimates. The results of pair-wise F ST and R ST calculations indicated significant ( p <0.05) and varied ( F ST 0.049 to 0.241; R ST 0.000 to 0.330) genetic structuring between all sampled populations in Central India ( Table S5 ). Within cluster F ST estimates were mostly lower, ranging from moderately low (0.048 to 0.062) to high (0.079 to 0.102), compared to pairwise estimates between different clusters (0.127 to 0.217).
(A) STRUCTURE results.
Eight putative migrants were identified in STRUCTURE ( Table 3 ). Of these, four individuals (D954, D955, D958, D1399) were identified with>80% migrant and cross-assignment probability to a single non-home cluster in the STRUCTURE analysis, carried out without prior population information. The remaining four samples represented individuals (D1843, D1892, D1297, D2058) that had weaker migrant probability ( P >0.5 to <0.7) and showed variable Q (0.289 to 0.758), and with the majority of samples having cluster memberships to more than one non-home locality.
https://doi.org/10.1371/journal.pone.0111207.t003
(B) GENECLASS results.
GENECLASS also identified eight individuals ( Table 3 ) as putative migrants ( P <0.01), with high log likelihood of cross-assignment ( L h /L max >2.0). Lowering the likelihood threshold ( L h /L max <2.0) yielded further six putative migrants ( P <0.05).
(C) BAYESASS results.
A total of fifteen individuals with likely immigrant or admixed ancestry were detected in the BAYESASS analysis ( Table 3 ). Of the fifteen total migrants, eleven individuals had high migrant cross-assignment probabilities ( P >0.8), and four individuals had intermediate migrant assignments ( P = 0.509 to 0.617). In general, the posterior probabilities of migrant assignment were higher in BAYESASS compared to the STRUCTURE analysis. Additionally, BAYESASS identified six other individuals (D1075, D1381, D1383, D1393, D1400, D1987) as potential migrants ( P = 0.509 to 0.926) that were not assigned as migrants by either STRUCTURE or GENECLASS.
(D) CERVUS results.
Identification of offspring-candidate parent pairs in CERVUS yielded parentage relationships in thirteen out of seventeen putative migrants ( Table 3 ). No evidence of likely parentage (or sibling) relationships in the offspring population was observed. The cross-assigned population in ten of the thirteen individuals matched the parentage assignment in CERVUS, which serves to further corroborate the results of the migrant assignments. Only three mismatches (D1075, D1393 and D1987) were observed between the parent populations identified by CERVUS and the population assignments depicted by the migrant analysis, but this could be due to low information in the data as opposed to incorrect migrant assignment. Except for negative LOD value in two pairs (D955–D1182, LOD = −0.36; D1400–D1168, LOD = −2.15), LOD scores were positive in the remaining eleven putative parent-offspring pairs. The relationship between a potential migrant sampled in Pench (D958) with a candidate parent from Kanha (D1205) was identified with>80% confidence in assignment (LOD = 5.32). LOD scores in remaining offspring-parent pairs were below the 80% confidence limit (<4.9).
The detection of migrants by the above methods yielded a total of seventeen individuals with putative immigrant ancestry ( Table 3 and Figure 3 ). Identical migrant assignment across all three programs was observed in seven individuals (D954, D955, D958, D1297, D1399, D1843, D1892), while there was equivocal assignment in the remaining ten individuals. Sex identification revealed 12 out of 75 males (16%) and 5 out of 84 females (6%) as individuals with immigrant ancestry in the entire area. Figure 3 shows the posterior distributions of individuals assigned to nonimmigrant (gen0), first (gen1) or second generation immigrant (gen2) ancestry states in GENECLASS, STRUCTURE and BAYESASS. All GENECLASS migrants with L h /L max >2.0 were classified as 100% first generation migrants. Two individuals (D955, D958) with>90% gen1 assignment and three individuals (D954, D1399, D1843) with relatively high gen1 assignment probability (0.5 to 0.7) were considered as migrants. Five individuals (D525, D1892, D2058, D1297 and D1987) showed moderate levels of migrant assignment and immigrant ancestry patterns are indicative of admixed status. The assignment status of seven more individuals (D1075, D1381, D1383, D1393, D1400, D1140 and D2154) was equivocal. While STRUCTURE could not assign them as migrants, they were identified as potential second generation migrants or admixed individuals in BAYESASS.
Posterior distributions of individual assignment to nonimmigrant (gen0), and first (gen1) and second generation immigrant (gen2) ancestry states. Suffixes after indvidual names indicate assignment probabilties as obtained in GENECLASS (G), STRUCTURE (S) and BAYESASS (B).
https://doi.org/10.1371/journal.pone.0111207.g003
Contemporary and Historical Migration Rates
The mean posterior distributions of pairwise immigration rates depicting contemporary gene flow estimates in BAYESASS are shown in Tables 4 and 5 . Most populations have low migrant proportions with the exception of migration from Pench to Melghat ( m = 0.09) and Kanha to Pench ( m = 0.07) where the rates were more than 5% ( Table 4 ). Gene flow between Melghat and Pench was likely asymmetric and there appears to be a source-sink relationship because the expected proportion of migrants into the Pench population from Melghat is much smaller ( m = 0.015). Asymmetric migration was also visible between the Pench and Kanha population clusters, as the proportion of migrants from Pench to Kanha was negligible ( m = 0.006). The Bandhavgarh population was devoid of migrants as suggested by the lack of gene flow between other populations ( m <0.01). As in the locality-wise analysis, similar asymmetry and rates of migration were obtained between the various STRUCTURE defined population clusters ( Table 5 ).
https://doi.org/10.1371/journal.pone.0111207.t004
https://doi.org/10.1371/journal.pone.0111207.t005
Results from the MIGRATE analysis showed low estimates of relative effective population size (theta, θ ) and historical mutation scaled immigration rate ( M ) suggesting very low overall migrant proportions in the area over the long-term ( Table 6 ). Theta estimates were low to moderate and ranged from 0.57 (Bandhavgarh), 0.77 (Kanha-Achanakmar, and Melghat-Satpura-Tadoba) to 1.5 (Pench). Estimates of M ranged from a high of 6.17 (Pench to Bandhavgarh) to a low of 0.9 (Bandhavgarh to Melghat-Satpura-Tadoba), revealing limited to no migration among populations in the landscape ( Table 6 ). Of the twelve pairwise population comparisons, ten pairs showed asymmetric migration patterns, with the higher value of M representing immigration from the population with the larger theta value (Kanha and Pench clusters), to the population with the smaller theta value (Melghat-Satpura-Tadoba and Bandhavgarh clusters). The number of migrants per generation ranged from almost zero (Bandhavgarh to Melghat-Satpura-Tadoba cluster) to nine (Pench to Bandhavgarh). The Pench and Kanha clusters represented the largest source populations for immigrants in the area. Though marginally higher migration from Pench to Kanha was visible compared to migration in the other direction, both population clusters had overall symmetric gene flow.
https://doi.org/10.1371/journal.pone.0111207.t006
Genetic Bottleneck
Except for Bandhvagarh, which had a low M ratio of 0.66, all other populations showed M values above the critical threshold of 0.68 ( Table 2 ). Wilcoxon's sign rank test of heterozygosity excess were not significant ( p >>0.05) regardless of the mutation models used and all localities tested showed normal L-shaped allele distributions in the mode-shift test, indicative of stable non-bottlenecked populations ( Table 2 ). Only, the Pench and Kanha populations showed significant ( p <0.05) evidence of heterozygosity deficiency ( Table S6 ), which could suggest recent events of expansion in these two populations.
Tiger Occupancy, Habitat, Prey and Human Disturbance
Ten Principal Components (PCs) had Eigen values greater than one and together explained 86.6% of the variability of the original variables ( Table 7 ). The factor loadings of the components permitted unambiguous ecological interpretation of PCs ( Table 7 ). The detection probability of tiger sign was 21.98% (SE 0.04). Detection of tigers was best explained by index of tiger abundance. The closest competing model for detection differed by a ΔAIC value of 1207 ( Table 8 ). Tiger occupancy was best explained by a model that included PC1 to PC9 and all the covariates conformed to the a priori predictions of their influence on tiger occupancy. The best model differed from the full model by ΔAIC of 0.45 and from the null model by ΔAIC of 1682. Tiger occupancy in a grid was best explained by (a) PC1 that represented availability of large undisturbed good canopy forests, (b) PC2 and PC4 that represented grids that had low human and livestock disturbances, (c) PC3, PC6 and PC9 that represented high ungulate prey especially in the form of chital, sambar, wild pig and gaur, (d) PC5 representing lower elevation, (e) PC7 representing grids within or in the proximity of legally protected areas and (f) PC8 that gave greater loadings for grids with higher rainfall ( Tables 7 and 8 ). The sign of the coefficients of the best model for each PC was the same when models were run using original variables in a univariate model ( Table S7 ). The goodness-of-fit test for observed data against 50,000 model based bootstrap samples failed to show lack of model fit (χ 2 P value = 0.11), Ĉ statistic was estimated at 1.4 and standard errors shown in Table 9 are corrected for over-dispersion by a factor of 1.18. Out of the 185,100 km 2 area of the sampled grids, 76,913 km 2 was forested or potential tiger habitat. The naïve estimate of grid occupancy was 17.5% while the model-averaged estimate of occupancy was 20.87% giving a 3.4% increment over the naïve estimate. The naïve estimate of tiger occupied habitat was 19,845 km 2 while the model inferred occupancy of forests was 21,290 km 2 giving an increment of 1.8% in occupancy estimate. The habitat variables of forest area, forest core area, rainfall, NDVI, and terrain ruggedness had significantly higher values in tiger occupied grids compared to unoccupied cells. All wild tiger prey indices were higher, while livestock abundance indices were lower in tiger occupied grids. Relevant human impact indices had significantly lower values in tiger occupied grids ( Table 9 and Figure S4 ).
https://doi.org/10.1371/journal.pone.0111207.t007
https://doi.org/10.1371/journal.pone.0111207.t008
https://doi.org/10.1371/journal.pone.0111207.t009
Tiger populations within the landscape were primarily located in and around tiger reserves ( Figure 1 ). The Pench-Kanha-Achanakmar tiger population was located in the largest patch of contiguous forest comprising 16,063 km 2 with intermittent tiger presence recorded throughout this patch even outside the legal reserve boundaries. The Satpura-Melghat forest patch was 12,720 km 2 , while the forest patches that contained Tadoba and Bandhavgarh Tiger reserves were smaller 2,088 km 2 and 1,902 km 2 respectively and connected to larger adjacent patches by fragmented forests ( Figures 1 and 4 ). Melghat Tiger Reserve had the largest critical core area that is legally mandated to be made free of human habitation ( Table 1 ). The total protected area in the landscape under the tiger reserve system was 13,054 km 2 with 6,395 km 2 as core area. Tiger population extent was largest for the Tadoba population at 3,519 km 2 , while the smallest area occupied (904 km 2 ) was recorded in Achanakmar ( Table 1 ).
Tiger movement modeled as current flow within the Central Indian Landscape using tiger occupancy probability and drainage systems as conductance layers and human settlements as high resistance barriers in CIRCUITSCAPE. Light colors indicate potential habitat corridors. Note the prominent bottlenecks observed in the Kanha-Pench, Kanha-Tadoba, and Tadoba-Melghat habitat corridors.
https://doi.org/10.1371/journal.pone.0111207.g004
Corridor models – maps and corridor cost values between source pairs
The least-cost corridor plot joining tiger reserves ( Figure 1 ) shows that the longest corridor was between Bandhavgarh and Melghat; and the shortest corridor was between Kanha and Achanakmar ( Table S8 ). The maximum number of barriers in the form of crossings of national highways was five for the corridor connecting Bandhavgarh to Melghat. CIRCUITSCAPE results detected bottlenecks in connectivity in corridors connecting Kanha with Pench, Tadoba with Kanha and Tadoba with Melghat ( Figure 4 ). Alternative habitat connectivity besides the least cost corridor was detected by CIRCUITSCAPE to exist between Bandhavgarh and Melghat along the sparse ridge forests on the southern banks of the Narmada river as well as between Tadoba and Melghat with patchy connectivity observed through Bor wildlife sanctuary. Habitat connectivity between Achanakmar and Bandhavgarh was diffused with no clearly defined flow pathways being observed ( Figure 4 ).
Genetic structure, migrants and corridor costs
Mantel's r correlations between pairwise genetic distances and spatial distance metrics were positive, and showed a similar trend across all three genetic distance estimators ( Table S9 ). The highest correlations were observed for IBR and LCC distances with genetic distance while geographical distances showed the lowest correlations with genetic distance. Linearized R ST had weak non-significant correlations with spatial distances, compared to F ST and Phi PT estimates. A significant linear relationship was observed between population pairwise F ST /(1− F ST ) genetic distances vs. resistance ( r = 0.549, p = 0.019) and least-cost corridor distances ( r = 0.533, p = 0.009). In comparison to Euclidean geographic distance correlations, this corresponded to a 29.5% and 25.7% increase in model fit for the circuit theory based IBR and the LCC models respectively. The proportion of migrants was higher between population pairs that had lower corridor costs ( Figure 5 ). Linearized Phi PT estimates also produced significant positive correlation with both least-cost corridor ( r = 0.416, p = 0.035) and resistance distances ( r = 0.462, p = 0.023). This meant a 46.2% and 31.6% increase over log-transformed Euclidean distance correlations for the circuit theory and corridor models respectively.
The size of each circle is representative of the proportion of migrants shared between each population pair. Depicted corridors are Kanha-Bandhavgarh (KB), Kanha-Achanakmar (KA), Pench-Kanha (PK), Melghat-Kanha (MK), Satpura-Kanha (SK), Satpura-Pench (SP), Melghat-Pench (MP), Melghat-Satpura (MS), Kanha-Tadoba (KT), Pench-Achanakmar (PA), Melghat-Achanakmar (MA), Achanakmar-Bandhavgarh (AB), Pench-Bandhavgarh (PB), Satpura-Bandhavgarh (SB) and Melghat-Bandhavgarh (MB).
https://doi.org/10.1371/journal.pone.0111207.g005
Correlations in partial Mantel tests showed a significant, positive relationship between genetic and resistance distances, after controlling for all competing spatial distance metrics (linearized F ST : r >0.48, p <0.02; linearized Phi PT : r >0.39, p <0.05). In contrast, after controlling the effect of resistance distance, significant positive correlation was only observed between linearized F ST vs. geographic and least-cost corridor distances, while partial correlations between all other spatial and genetic distance metrics were either non-significant or negative. Although significant positive correlation was observed between F ST and geographic distances in the standard Mantel tests, genetic and geographic distances were uncorrelated in the partial Mantel tests, after controlling the effect of population clusters, thus affirming that isolation-by-distance (IBD) pattern was absent in the data. Only resistance (IBR) and LCC distances retained a significant, positive partial correlation with genetic distances, when controlled for the effect of population clusters (linearized F ST : r >0.4, p <0.03; linearized Phi PT : r >0.4, p <0.04).
Genetic diversity and population structure
The present study is illustrative of the general strengths and challenges in using non-invasive genetic samples to assess spatial distribution patterns in an endangered and cryptic species living in fragmented habitats. Although maternally inherited mitochondrial DNA could elucidate patterns of female gene flow, the sequencing of mitochondrial genes was not attempted as the primary objective of the study was to assess the functionality of corridors through the detection of first and second generation migrants. Hence, highly polymorphic, bi-parentally inherited, co-dominant and selectively neutral nuclear loci such as microsatellites were most suited for this purpose. Microsatellite marker amplification success was low from scats, reflecting the often poor quality of samples, typical of tropical environments [127] . Even though standardized methods of sample preservation and DNA extraction were used for the study, finding fresh scats (which may have improved results) was rare despite repeated surveys, due to the low tiger density of many sampled areas, the remoteness of the terrain, and further compounded by the heat and humidity of the region which enhanced degradation of scats. Despite these constraints a relatively sizeable sampling was achieved with the total number of identified individuals ( n = 169) representing about 49% of the estimated population [31] in the entire landscape ( Table 1 ). The gender proportions observed in our data are similar to the estimates obtained from camera-trap studies in the area [31] . The relatively large sample size in relation to the estimated population enhances the significance of our results.
Most individuals genotyped in this study, possessed heterozygous genotypes at>50% of typed loci, and there was no discernible differences in heterozygosity between migrant and resident individuals ( Figure S2 ). However, unlike resident individuals, we found no migrants with fewer than five heterozygous loci, or completely heterozygous across all eleven loci. Eleven of the seventeen identified migrant individuals were slightly skewed towards a higher heterozygosity distribution, being heterozygous at eight to ten loci, while the rest of the migrants were polymorphic at five to seven loci. This could be due to the contribution by second generation migrant individuals which constituted the bulk of migrant pool. These individuals are more likely to possess a higher number of heterozygous loci than resident tigers, since they are admixed with parental genotypes from different allelic distributions.
The allelic diversity (9.1±2.2) and heterozygosity ( Ho = 0.701±0.059, He = 0.754±0.039) observed in our study is typical of genetic diversity prevalent among tigers in the Indian subcontinent [23] , [128] , [129] . Related studies, using different microsatellite markers than the ones used here, have observed levels of average expected heterozygosity ( He ) and mean number of alleles ( A ) to be lower in other subspecies, viz. P. t. altaica ( He = 0.26±0.11, k = 2.6±0.84 [130] ), P. t. sumatrae ( He = 0.493±0.039, A = 3.60±1.48 [129] ), P. t. jacksoni ( He = 0.571±0.027, A = 3.90±1.18 [129] ) and P. t. corbetti ( He = 0.670±0.027, A = 6.03±1.81 [129] ). Interestingly, allele diversity and heterozygosity in the Indian tiger was comparable to other felid species [129] , such as the jaguar ( P. onca , He = 0.792±0.0137, A = 8.67±1.72 [131] ), Asian leopard ( P. pardus , He = 0.790±0.0174, A = 10.71±2.31 [129] ), African lion ( P. leo , He = 0.610±0.0348, A = 5.0±1.75 [129] ), South American puma ( Puma concolor , He = 0.774±0.0247, A = 7.0±1.76 [129] ), and cheetah ( Acinonyx jubatus , He = 0.708±0.130, A = 6.1±1.8 [132] ). Although not directly comparable with the present study because of the different markers used, a recent study in the Satpura-Maikal landscape in Central India [64] based on seven microsatellite loci, detected similar levels of heterozygosity ( Ho = 0.65±0.09, He = 0.80±0.05), and allelic diversity ( A = 7.76±1.96), and very low genetic subdivision (mean F ST = 0.013±0.006). Another study in the southern parts of the Central Indian landscape, focused primarily in peripheral habitats just outside the scope of this study area [66] also observed high mean heterozygosity ( Ho = 0.54, He = 0.81), and allelic diversity ( A = 11.71) levels at fourteen microsatellite loci. A range-wide study [23] conducted with five microsatellite loci showed that Indian tigers have higher heterozygosity ( Ho = 0.70±0.16) and allelic diversity ( A = 12.4±3.6) compared to all other tiger subspecies ( Ho = 0.53±0.07, A = 7.2±1.2). The study also reported low and non-significant genetic structuring of the Central Indian tigers with the Northern ( F ST = 0.027, p = 0.063) and Southern Indian populations ( F ST = 0.019, p = 0.054). This could be attributed to a historically large effective population size and inter-population connectivity in the region of Central and Peninsular India, explaining why, despite centuries of immense trophy hunting and continued habitat fragmentation, extant tiger populations in the region currently retain close to 60% of the global genetic variation in the species [23] .
By using a combination of classical population differentiation and individual clustering approaches, we were able to detect patterns of population sub-structuring in the region. Results from both the PCoA and STRUCTURE analyses suggest the presence of four genetic clusters in the area. The clustered localities occur in close proximity to each other and the overall pattern of genetic structuring observed in the landscape is concordant with existing habitat connectivity and indicative of the role that habitat fragmentation plays in shaping the distribution of allele frequencies across populations. Significant genetic structuring was also detected in the population pairwise F ST and R ST statistics, which may have biased parameter estimations in the STRUCTURE runs carried out without prior population information (locprior = 0) resulting in higher hierarchical levels of population subdivision at K = 8. In contrast, the runs carried out with population information (locprior = 1, K = 4) did not detect unnecessary genetic structuring, and ignored the prior sampling location information if the ancestry of individuals was uncorrelated [126] . The cluster solution of K = 4 appeared optimal for the following reasons. In cases where STRUCTURE detects multiple clustering options with similar probabilities, typically the lowest K value which captures much of the biological complexity in the sample is considered to be the most conservative [126] . Additionally, the presence of related individuals in our sample and the model of correlated allele frequencies used for analysis can lead to overestimation of the true K value [126] . Variation across the four STRUCTURE-defined population clusters was weak, but significant, in the AMOVA test, as the major portion of genetic variance was attributed to within population variation. Though the AMOVA results and F ST statistics indicated significant pairwise structuring across all populations in the area, the pattern shared certain similarities to the population clusters identified by STRUCTURE and the ordination results in PCoA. F ST values were generally lower for localities within the same cluster compared to pairwise estimates between different clusters. We therefore treated the genetic distance estimators including F ST and its analogues as relative measures of population differentiation. The estimation of these parameters requires prior identification of populations and unless the population units are clearly known, such a priori designation may not reflect realistic biological patterns as they would only be representing ad hoc division of populations [133] . Importantly, the assumptions of demographic and genetic equilibrium along with long time scales under which F ST and its analogues are based may not be suited for estimating genetic differences between populations that have undergone fragmentation or demographic fluctuation events only recently. On the other hand, the results of the model based STRUCTURE clustering approach, which partitioned individuals into relatively distinct clusters based on iterative assignments, made much more sense of the biological realities of the area as the structuring observed here is likely an artifact of recent population fragmentation.
Additionally, the differences observed between F ST and R ST values can provide valuable insights into the balance between genetic drift and mutation events in the studied populations [134] . In this study, pairwise genetic differences between tiger populations in the landscape showed higher F ST values compared to R ST values. The F ST statistic is based on allelic identity and accounts for gene flow between different populations as the basic premise under which it estimates pairwise genetic differences [134] . In contrast, R ST relies on allele size and single stepwise mutations are the primary contributors of genetic variation for this statistic [134] . R ST estimations produced non-significant results across many pairwise comparisons, and had higher sampling variances compared to F ST . This suggests that populations in the area were not isolated long enough for mutations to have caused the genetic differences between populations and the primary cause of genetic structuring in the area is due to recent genetic drift. Some measure of this drift is evident from the number of private alleles found in each population, especially Bandhavgarh, which has the highest individual allele frequency compared to all other localities ( Table S3 ).
Although the STRUCTURE and PCoA results suggest subdivision at four clusters for the Central Indian tiger population, the significant F ST structuring observed between all populations could also be indicative of ongoing fine-scale genetic differentiation in the area. Existing patterns of population structuring are a result of past fragmentation effects and fragmentation is not a static process. Gene flow in the area is currently meager and likely to become even lower, due to continued habitat loss and burgeoning anthropogenic activity in the area. Many localities still retain marginal inter-population connectivity, as evidenced by the presence of individuals having immigrant ancestry and further substantiated by camera trapping and radio-telemetry. However, genetic isolation of almost all populations in the foreseeable future is likely if current patterns of habitat fragmentation persist. In the case of Bandhavgarh the extent of fragmentation appears to be so great that the population may have already become genetically isolated for a longer period than the other reserves. Efforts should be made to revitalize the least cost corridor connecting Bandhavgarh with Achanakmar and subsequently to the gene pool of the main Central Indian complex by a combination of restorative ecology and legal instruments (See conservation implications below).
Migration in the Central Indian tiger population
The Central Indian landscape is a mosaic of habitats where tiger population densities [31] varying from high (Bandhavgarh 14 tigers/100 km 2 ) to low (Achanakmar 0.1 tigers/100 km 2 ) are juxtaposed in a matrix encompassing a range of land-use regimes from undisturbed natural forest to high density human settlements ( Figure 1 ). Despite being one of the most fragmented TCL in India [31] , some potential for gene flow across populations within the landscape exist because tigers, like most large carnivores, have the ability to disperse across great distances where habitat connectivity is present (e.g., ∼150 km linear distance between two reserves in Nepal [67] ). However, in the fragmented human-dominated Terai Arc landscape of Nepal, tigers were not found to disperse across expanses of agricultural land (10 to 20 km wide) though they did traverse through stretches of degraded forest [41] . So far, the only published dispersal estimates from the Central Indian landscape are from recent genetic studies that observed first generation migration and long-range dispersal across protected areas located roughly 200 km [65] and 650 km [66] apart, suggesting that tigers may be more resilient in traversing fragmented habitats than previously reported. Extensive annual camera-trapping since 2006 has recorded tigers dispersing between Pench and Kanha, a geographic distance of more than 150 km (Jhala and Qureshi unpublished data).
Identification of migrants by the methods used in our study requires that the populations being investigated have sufficient genetic separation and low levels of migration. We found in our study that the power to detect migrant individuals varied across localities in the region. There was no power to detect migration between localities in the same cluster (such as Kanha-Achanakmar). In these situations it was difficult to tease apart actual migration events from similar allelic patterns that arose from shared population histories and low genetic separation. On the other hand, migrant identification between localities in different population clusters produced robust assignments because of distinct genetic differences. Migrant detection was highest between localities that had discernible genetic separation and had relatively intact habitat corridors with confirmed tiger presence, such as between Kanha and Pench populations where four migrants and seven likely admixed individuals were detected. Other studies by Joshi et al. [66] and Sharma et al. [65] , respectively, detected one and two first generation migrants between Pench and Kanha. The intervening forest patch between Kanha and Pench not only served as a movement corridor but also had some resident tigers as evidenced from camera trap data [135] . A noninvasive genetics study by Sharma et al. [64] detected seventeen individuals from this corridor. At the extreme end in Bandhavgarh, no migrants were detected even though distinct genetic structuring of Bandhavgarh from other population clusters provided sufficient power to distinguish migrants. Between the Melghat and Pench populations where three individuals with migrant ancestry were obtained, there was limited but adequate power to detect migrants as genetic separation was distinct but at a lower level than Bandhavgarh. One individual sampled in Satpura was cross-assigned to Pench. The corridor between Pench and Satpura has fragmented forest connectivity and tigers were reported from this area within the last ten years [42] . Two individuals with admixed ancestry were also detected between Kanha and Tadoba, though one of these had mixed assignments with Pench as well suggesting gene flow in the last few generations. Two individuals with admixed ancestry were also detected between Kanha and Tadoba. Areas of degraded forest and low density tiger occupancy such as Nagzira and Nawegaon Wildlife Sanctuaries located strategically between Tadoba and Kanha, and Bor located between Tadoba, Pench and Melghat may serve to provide stepping stone [136] type corridor connectivity ( Figure 1 ). These areas have resident tigers, and it seems likely that the populations are sustained by sporadic dispersing individuals from larger source populations. Joshi et al. [66] observed that Tadoba, Nagzira and Melghat form a genetic cluster, and based on asymmetric migration patterns in their study it appears that the tiger population in Nagzira acts as a sink population for the high density source population in Tadoba.
Based on our strict criteria of defining migrants, we designated four individuals, three males (D954, D955 and D1399) and one female (D958) that had high cross-assignments ( Q >0.8) to a single non-home cluster, as first generation migrants. Further, a fourth male individual (D1853) that had high cross-assignments, albeit with marginally lower Q value (0.527) compared to the above four migrants, was also designated as a first generation migrant. The immigrant ancestry status in the remaining ten putative migrant individuals was classified as admixed based on - (i) intermediate levels of migrant probability in STRUCTURE; (ii) membership to more than one cluster in the individual Q assignments; and (iii) high probability of assignment to second and not to first generation immigrant ancestry state in STRUCTURE and BAYESASS.
Though one of the first generation migrants was a female, most individuals with immigrant ancestry were males (80%: 4 males out of 5 total migrants), confirming that dispersal is male-biased in tigers [41] . The low number of strict first generation migrants suggests that contemporary migration events are minor in proportion to the number of individuals with likely admixed ancestry. The identification of samples with admixed ancestry suggests that most migration events in this landscape have occurred within a few generations and is evidence that migrant tigers are able to reproduce in the new locality. This genetic evidence is further supported by field observations where a sub-adult male tiger photo-captured in Pench tiger reserve in 2006 was observed to be a territorial breeding male in Kanha tiger reserve in 2010 (Jhala and Qureshi unpublished data). The data and analyses show that tigers likely disperse between Kanha-Pench, Pench-Satpura-Melghat, Kanha-Tadoba, Melghat-Tadoba and Tadoba-Pench.
Gene flow rates and past demography
Both the contemporary and historical analyses detected low estimates of migration rate between populations in the landscape. While this result is superficially similar to the findings of Joshi et al. [66] , it is in sharp contrast to Sharma et al. [65] where they found both high historical and contemporary gene flow, although historical rates were much higher than contemporary rates. As observed in our study and by Joshi et al. [66] , low levels of contemporary gene flow are expected given that the area is highly fragmented and extant populations occupy habitats that are completely surrounded by heavily modified landscapes, altered by agriculture and high density human settlements, thus making it difficult for tigers to disperse between populations. The highest estimate of contemporary migration was seen from Pench to Melghat, but there appears to be an asymmetric source-sink relationship between the two populations with very low migration from Melghat to Pench. Such a pattern is expected given that Pench has high tiger density (4 tigers/100 km 2 ) likely serving as a source population, while the population density in Melghat is lower (2 tigers/100 km 2 ). Asymmetric contemporary rates of gene flow were also apparent between Pench and Kanha, as migration from Kanha (6 tigers/100 km 2 ) to Pench was much higher compared to movement in the opposite direction from Pench to Kanha. Joshi et al. [66] similarly detected high gene flow (>5%) between Kanha and Pench, with Kanha acting as the biggest contemporary source for immigrants. In contrast, Sharma et al. [65] state that only Pench is acting as a contemporary source population and contemporary gene flow from Pench to Kanha and Satpura is very high, and has remained relatively stable since historic pre-disturbance times. A comparative evaluation at historical and contemporary time scales in their study [65] showed that gene flow between the Pench–Satpura and Melghat–Satpura pairs remains similar, whereas there has been a 47–70% reduction in gene flow between Kanha–Satpura, Pench–Melghat and Kanha–Melghat. Our results showed that historical patterns of migration between the two major population clusters of Kanha and Pench were of equal, albeit low proportion in both directions, and that both Kanha and Pench acted as source populations in contemporary times as well. Although Sharma et al. [65] reported higher historic and contemporary gene flow rates compared to our study, both studies similarly observed that Kanha and Pench were important historic source populations and were the main drivers of gene flow in the area.
Contemporary density estimation studies using photographic capture-recapture techniques in the region [31] help explain some of the patterns observed in the gene flow analysis. During 2006, the Kanha population experienced a phase of relative expansion compared to a decline observed later in 2010. The situation was reversed in Pench where the population was relatively low during 2006–2009 compared to a later phase of expansion in 2010 [31] , [42] . From the genetic sampling done in Pench during both 2007 and 2010, which pre and post-dated this period of population expansion in the locality, a range of individuals representing likely migrant or admixed ancestry to Kanha was obtained. All first generation migrants from Kanha to Pench were detected during 2007 only, when the population in Kanha was in expansion phase. In 2010, seven individuals showing admixed ancestry from Kanha were obtained in Pench. Dispersal and subsequent breeding by immigrants from Kanha to Pench during periods of population expansion in the former appears to explain the patterns of immigrant ancestry detected in the Pench population.
As parameter estimates of migration rate and theta in MIGRATE average across at least 4 Ne past generations, [137] or several thousands of years ago in absolute time, the low long-term gene flow estimates observed in this study could suggest that populations may have been subject to historical fragmentation and genetic drift. Although ancient population fragmentation is perceived to have occurred in response to the forest clearing activity of agro-pastoralist Neolithic people during the mid-Holocene about 5,000–3,000 years ago [138] , the effect is difficult to evaluate as early farmers likely did not clear forests at a scale comparable to recent centuries [139] . However, it is conceivable that the shift from hunter-gatherer to agro-pastoralist lifestyle and successful colonization of new areas would have negatively impacted large mammal populations including tigers and prey species through hunting (and reduction of prey base), as seen during human colonization events in other parts of the world [140] , [141] . Evidence from an exhaustive range-wide study of tigers has detected a massive decline of about 98% in the number of tigers over the last 200 years in peninsular India [23] . The wanton slaughter of tigers and other wildlife can be glimpsed from historical hunting records of the area, where upwards of 1,000 tigers and 2,000 leopards from this region were killed within just a few decades [25] . The population decline continues apace today, as omnipresent fragmentation and rampant poaching of tigers, other carnivores and prey species have reduced tiger populations such as at Achanakmar to only a few individuals [31] , and resulted in their local extirpation in adjacent reserves of Sariska [5] and Panna [29] .
Alternatively, the low estimates of historic migration rate raise concerns whether these results are artifacts of sampling or population related. MIGRATE parameter estimations assume coalescent-based models of constant population sizes and mutation-migration-drift equilibrium. Departures from the equilibrium model such as recent and sudden declines in population sizes can negatively bias the posterior parameter distributions of theta and hence migration rate estimations [137] . However, this study and a recent work by Sharma et al. [64] did not find significant evidence of past demographic contraction. In our study, only the population of Bandhavgarh had below par M ratios (<0.68) suggestive of bottleneck, but the evidence was equivocal since no significant heterozygosity excess or a mode-shift in allele frequencies was detected. Though bottlenecks were not observed in this study, the analyses may be undermined in a few populations because of low sample sizes (<20 individuals), as tests such as the mode-shift in allele frequencies is known to be affected by sample size variances [106] . Also, a demographic decline may not necessarily result in a bottleneck as several factors such as duration of decline, pre-bottleneck diversity, and gene flow between populations can affect the probability of detecting a bottleneck [101] , [106] , [142] , [143] . Furthermore, the significant heterozygosity deficit (symptomatic of recent population expansion) observed in the Pench and Kanha populations could mask signatures of population bottleneck as the addition of new individuals could increase the number of rare alleles which can bias allelic and heterozygosity distributions [106] . Demographic expansion in Pench and Kanha is likely, given the recent history of tiger population recovery in the area in the 1970s–1980s [28] , [144] , or about six to eight generations ago (considering a five year generation time in tigers [99] ). This period is well within the window of detection for genetic bottleneck tests of heterozygosity deficiency/excess which assess short term demographic changes only, maximum up to ten generations before present [143] . We could not account for other genetic effects or demographic events which could affect the heterozygosity distributions in these two populations, although our analysis did detect some amount of higher hierarchical STRUCTURE clustering patterns which could be indicative of lineage or extended family structure, since the data definitely contained related individuals. However, even if kin-based segregation did produce a signature of heterozygosity deficit, the specific nature of the effect is difficult to evaluate without in-depth parentage and relatedness analyses, and backed by field investigations, which was beyond the scope of this study. Based on both the theta estimates and absence of bottlenecks, our results suggest that the bigger source populations in the study area have had a relatively stable population history, compared to the smaller populations, as also observed by Sharma et al. [65] .
Our study and the recent study by Joshi et al. [66] had higher power to resolve hierarchical genetic structuring in the area compared to Sharma et al. [65] where indistinct structuring was observed. This was most likely due to the higher number of loci used in both studies compared to Sharma et al. [65] . Except for Tadoba where sampling was low due to logistic constraints, the small sample sizes from some other sites were due to small tiger populations. Simulation studies by Paetkau et al. [94] caution regarding the use of MCMC resampling methods implemented in Rannala and Mountain [95] , as they tend to over-estimate migrants from a limited data set. In our case, this translates to the possibility that there may be less migration than we report in some of the smaller populations in the study system. Due to low sample sizes, individuals from Achanakmar (n = 4) and the Kanha-Pench corridor (n = 5) were not analyzed separately, as doing so resulted in overestimation of migration rates. Instead these localities were merged with the Kanha cluster, following the results of STRUCTURE assignments. Low sampling was also a problem in the study of Joshi et al. [66] as sites such as Kanha, Pench and Melghat were clearly under-sampled.
Occupancy has often been used as a straightforward and economical state variable in place of abundance to monitor populations [145] . Occupancy of tigers has been estimated in the Mysore-Wayanad Landscape [115] and the Corbett-Rajaji Landscape [116] and across three major tiger landscapes of India [22] . Correcting for detection bias by replicate surveys within sampling units is especially important when detection probabilities are low, sample sizes and survey lengths are small resulting in naïve occupancy estimates that are substantially negatively biased [115] . The recent availability of powerful analytical tools and software [70] , [114] that permit accounting for bias caused by detections being <1 has promoted the use of correcting for such bias post data collection [115] , [146] compared to more rigorous designed field methods that minimize such bias in the first place. In this study we use independent spatial replicates, each of 5 km in length, with a minimum effort of one survey for every 5 km 2 of tiger habitat. Thus a 10×10 km 2 grid had anywhere between 3 to 35 replicate surveys depending on the amount of tiger habitat present within that grid. The detection probability of tiger sign was high in comparison to other studies [115] due to longer spatially independent surveys as well as higher density of surveys. Therefore, the difference between naïve and bias corrected estimates of occupancy were comparatively small (3.4%). Several assumptions that are difficult to meet underlie the use of occupancy as a state variable to monitor populations [147] . Further, tiger populations are at risk of poaching and they can be severely reduced or even extirpated from prime habitats [5] , [29] . Thus, model inferred occupancy from covariates of habitat, prey, and human disturbance can provide misleading inference regarding true occupancy in the case of tigers and other species that can be severely depleted by poaching, and should therefore be interpreted with caution. However, occupancy models serve to provide a good assessment of habitat suitability at a scale relevant for conservation management [70] , [146] . It is in this context that we primarily use the occupancy probability in this paper so as to model habitat corridors joining tiger populations.
Detection of tiger sign was better modeled by including encounter rate of sign per km walk. Intensity of sign was found to be a reliable index of tiger abundance [22] . Detection of sign was likely to be higher with greater abundance of tigers and this was reflected in our model. Principal Components that represented distance from protected areas, prey abundance and remote canopied forests had the highest coefficients in the logit-link function that modeled tiger occupancy. All the model coefficients were in concordance with the a priori predictions based on our understanding of tiger ecology. Since PCs are orthogonal to each other [117] coefficients can be interpreted in terms of their sign and magnitude as the models are free from the effects of multicollinearity. The model coefficients of all site variables when used independently to model tiger occupancy as univariate models also conformed to the a priori predicted effects and to the coefficients obtained by the multivariate best model obtained from their PCs by PRESENCE. These results indicate that tigers are a conservation-dependent species, requiring areas having effective legal protection, good forest cover with least human impacts and high prey availability.
Corridor models, genetic structure, migrants and dispersal costs
Although both LCP and circuit theory used tiger occupancy probability as a base layer to parameterize landscape resistance surfaces, LCP defines the optimal minimal route that is required to connect two tiger reserves [53] , while the resistance layers obtained from circuit theory analysis provides an understanding of all habitat connectivity currently available between tiger reserves [118] . Circuit theory is especially important to identify bottlenecks in corridors where current is constrained to flow through a narrow channel due to high resistance of the neighboring matrix. Such bottlenecks are highlighted in Figure 4 and exist in almost all corridors connecting tiger reserves. A corridor is as good as its weakest link and if the high resistance habitat matrix surrounding these bottlenecks increase in their extent they could choke the corridor, thus forming a barrier to tiger dispersal.
In our study, the ecological distances that were generated using information from habitat heterogeneity and landscape resistance surfaces (LCCD and RD), correlated significantly with pairwise genetic distances, which validated the effectiveness of the modeled linkages in representing realistic biological scenarios. In particular, IBR models depicted well the ecological costs of movement as they accounted for multiple pathways, irregular patch effects, landscape heterogeneity and wider habitat swaths connecting populations [54] . Although the raw LCP model accounted for habitat heterogeneity while selecting a single pathway as the optimal minimal corridor, it did not correlate significantly with pairwise genetic distances (except F ST ). Instead, the LCCs which were delineated by matching and buffering the pathway results from the raw LCP model with existing ground forest cover maps of the area provided a more realistic relationship of the ecological costs with observed genetic differences. In contrast, Euclidean distances (GGD and log 10 GGD) did not correlate significantly with genetic distances, due to the null model assumptions of spatial homogeneity where habitats are arrayed in an infinite lattice [124] , and hence do not conform to the ground realties of fragmentation in the Central Indian landscape. The apparent IBD pattern observed due to the significant correlation of F ST (but not Phi PT or R ST ) with geographic distance is an artifact of metapopulation structure and discrete population clusters present in the area. This observation was affirmed by non-significant partial correlations between genetic vs. geographic distances (but not LCCD and RD) using the STRUCTURE identified population clusters as a control. Apart from fragmentation induced spatial heterogeneity, genetic structure is also a result of population history.
The results of our study imply that population subdivision and genetic structure across most localities in the area was strongly associated with habitat features that offer resistance to dispersal at different intensities such as agricultural land, roads, railway lines, high density human settlements and urban infrastructure and not only by geographic distance between populations. Mantel tests showed significant positive correlation of genetic differentiation with least-cost corridors and landscape resistance surfaces associated in traversing corridors, further confirming this observation. The tiger habitats in the region are patchy with some populations still having connectivity, while being reduced or conspicuously absent in others. The best patches of contiguous forested habitat are present in the corridor between Pench and Kanha, which extends eastward to Achanakmar. Likewise, the Satpura and Melghat populations are also connected through swathes of degraded forests, which are interspersed with agricultural land and medium density human settlements. Connectivity between Pench and Satpura is fragile as parts of the linkage are disrupted by mining activities, and broken up in places by agriculture, habitations, major highways and railway lines. The population in Tadoba is linked with Kanha in a stepping stone connectivity through patchily distributed forests. Even though the intervening matrix between Tadoba, Pench and Melghat is heavily human dominated, the populations are tenuously linked by degraded forest patches and tiger occupied habitats such as Bor Wildlife Sanctuary. Bandhavgarh has linkages with forest habitat and tiger reserves further east [31] , but seems isolated from tiger populations in the study area by human settlements and agricultural land. Corridors identified herein need to be given legal status, and mitigated with appropriate green infrastructure [148] for development projects within corridor habitats so as to ensure continued gene flow between populations.
Conservation implications
It is indeed surprising that in spite of being highly fragmented [31] , the tiger habitats in Central India still exist as a metapopulation with gene flow occurring between most population clusters in contemporary times. Similar conclusions were also reached in other studies [65] , [66] , which observed indistinct genetic structuring and low migration amongst populations, although the genetic differences were not substantial to permit unambiguous identification of migrants. These findings suggest that tigers are able to disperse through suboptimal habitat fragments, than was earlier believed [41] , [149] . The results of our study underscore the importance of conserving and maintaining corridor connectivity for the continued persistence of tiger populations in the landscape. We found seventeen individuals or about 10% of the sampled tigers with migrant ancestry. As revealed from this study, the sizes of genetic population clusters are clearly beyond the boundaries of protected areas, and have to be managed in a metapopulation framework stressing the need for a landscape conservation policy in place of the current conservation policy focused on protected areas. The functionality of corridors as shown in this study has important implications for the persistence of small populations such as Achanakmar, Satpura and Melghat by the rescue effect of emigrants from the large source populations in the landscape. Apart from poaching, the ecological isolation from other source populations, due to the absence of corridors was one of the prominent causes that led to the local extinction of tigers from the nearby protected areas of Panna and Sariska [5] , [29] . Now both of these areas have been repopulated through translocation of tigers from nearby reserves. A range of anthropogenic pressures are fragmenting important habitat corridors such as the ones linking Satpura with Pench, Pench with Kanha, Kanha with Tadoba, and Tadoba with Melghat. Dispersing tigers often have to negotiate passage through suboptimal prey-poor habitats and spend extended periods of time in proximity to human habitations, which greatly reduces survival and successful gene flow. The limited migration occurring in the landscape is probably due to tigers avoiding areas of human activity and high mortality due to commercial poaching or retributive killing in response to predation on livestock and humans. The eventual loss of genetic connectivity between populations in the near future, as observed currently in Bandhavgarh, is likely for most populations in the area if present trends of fragmentation continue unabated. In such situations, last resort alternative strategies such as translocation may be considered, as it could easily enrich genetic diversity by moving tigers across each of the four distinct genetic population clusters, to mimic natural patterns of gene flow. However, maintaining and restoring habitat corridors is the preferred conservation strategy to maintain genetic exchange between tiger populations, since corridors would serve the same function for other biota as well, exemplifying the role of the tiger as a flagship species.
Conservation efforts in such a fragmented and dynamic human-dominated landscape presents enormous challenges, but should be attempted in all areas where substantial habitat is still present. Our study gives renewed hope to tiger conservation efforts within the Central Indian Landscape and similar habitats with small fragmented tiger populations. We show that minimal habitat connectivity permits gene flow between populations, which is essential to maintain metapopulation connectivity. Our findings suggest that tigers could negotiate passage through stepping stone dispersal [136] as observed between Kanha-Tadoba, and Pench-Melghat. The most functional corridor was observed between Kanha and Pench, which has evidence of prey and offers the possibility of resident tigers in some of its larger forest patches [31] , [135] . However, infrastructural development in the form of adding lanes to national highways and widening of railway lines, threaten to form permanent barriers even within this corridor unless proper safeguards and mitigation measures [44] are built into these development projects. Tiger range countries like India are heavily investing in infrastructure development and mining to meet the needs of a growing economy [148] . Identification of minimal habitat corridors is vital for conservation efforts of tigers and other wide-ranging fauna like elephants ( Elephas maximus ), gaurs ( Bos gaurus ), leopards ( Panthera pardus ), and dholes ( Cuon alpinus ). They need to be offered legal protection with smart green infrastructure [148] being the norm of development policies within these corridor habitats. The extinction (nearly two decades ago) of gaur in Bandhavgarh, preceding their recent reintroduction with individuals from Kanha [150] , is symptomatic of habitat fragmentation events that have impaired movement with other source populations in the landscape. We demonstrate here an integrated approach to generate reliable information to document metapopulation structure of tigers and the required habitat connectivity [17] to maintain it in Central India. Legal mechanisms to safeguard these minimal corridors could potentially be the eco-sensitive category under the Environment Protection Act (1986) legislation. Currently tigers and other mega-fauna exist as a metapopulation and do exchange genetic material through functional corridors [65] , [66] , [128] , [151] . Such opportunities are likely to be lost rapidly in the wake of new wave of development unless legal sanctity, active restoration and mitigation of development projects become the norm. Conservation policy needs to shift the focus from protected area centered preservation to landscape scale conservation where development policies incorporate a conservation ethic.
Supporting Information
PCR-RFLP identification of tiger scats. ( A ) MtDNA cyt b alignment with GenBank and reference sequences showing polymorphism at the particular Bam HI restriction enzyme between tiger and leopard. ( B ) Enzyme digested bands of the 187 bp PCR product, targeting this region, showing different profiles in tiger and leopard for species identification.
https://doi.org/10.1371/journal.pone.0111207.s001
Frequency of heterozygous genotypes observed at each individual multilocus genotype in all tiger individuals (n = 169) in this study.
https://doi.org/10.1371/journal.pone.0111207.s002
Results of STRUCTURE analysis. ( A ). Difference in delta K and mean LnP(K) for an estimated number of K populations, in models run with (locprior = 1) and without (locprior = 0) prior sampling location information. ( B ). Summary barplots depicting prior and non-prior STRUCTURE runs (assumed K = 2 to 8), of sampled populations in central India showing cluster affiliations according to individual Q values. Cluster saturation at K = 4, indicative of four population clusters, is observed in runs carried out both with and without a priori location information. At K >4, increased sub-structuring is detected, but there is no concordance in clustering between the prior and non-prior runs.
https://doi.org/10.1371/journal.pone.0111207.s003
Differences in tiger present (n = 311) and tiger absent (n = 1540), 10×10 km grids shown as violin plots in the Central Indian Landscape. All variables are normalized by z transformation to make the scales comparable.
https://doi.org/10.1371/journal.pone.0111207.s004
Information on the 11 microsatellite loci used in this study. Allele diversity statistics, observed ( Ho ) and expected ( He ) heterozygosity, Hardy-Weinberg equilibrium (HWE) tests, null allele frequencies and sibling probability of identity ( PI-sib ) values obtained across 169 tiger individuals. Null allele frequencies>+0.05 are italicized.
https://doi.org/10.1371/journal.pone.0111207.s005
Summary of population cluster-wise genetic diversity statistics at each locus. Depicted are number of alleles ( k ), number of individuals typed ( N ), observed ( Ho ) and expected ( He ) heterozygosity, Hardy-Weinberg equilibrium (HWE) test significance and null allele frequencies ( Null ). The Achanakmar (n = 4) and Kanha-Pench corridor (n = 5) individuals are included in the Kanha cluster.
https://doi.org/10.1371/journal.pone.0111207.s006
List of diagnostic alleles present in the sampled populations.
https://doi.org/10.1371/journal.pone.0111207.s007
AMOVA results.
https://doi.org/10.1371/journal.pone.0111207.s008
Population pair-wise F ST (below diagonal) and R ST (above diagonal) estimates.
https://doi.org/10.1371/journal.pone.0111207.s009
Bottleneck test results for loci under different mutation models. P values <0.05 are depicted in italics.
https://doi.org/10.1371/journal.pone.0111207.s010
Variable attributes used as site covariates in modeling tiger occupancy.
https://doi.org/10.1371/journal.pone.0111207.s011
Habitat corridors, major roads, corridor cost between tiger reserves.
https://doi.org/10.1371/journal.pone.0111207.s012
Results of standard and partial Mantel tests for correlation between pairwise genetic and spatial distance metrics. The correlation coefficient ( r ) and probability ( p ) are shown using three different genetic distance estimators. Significant values ( p <0.05) are indicated by an asterisk (*).
https://doi.org/10.1371/journal.pone.0111207.s013
Details of cyt b PCR amplification in reference samples using felid-specific (187 bp) and universal primers (309 bp). + indicates all samples which amplified. NA – not amplified.
https://doi.org/10.1371/journal.pone.0111207.s014
Information on the pilot test carried out on scats ( n = 65) for species identification of tiger samples by PCR and Bam HI restriction enzyme digestion.
https://doi.org/10.1371/journal.pone.0111207.s015
Species identification from scat samples.
https://doi.org/10.1371/journal.pone.0111207.s016
Generating the cost surface for PATHMATRIX and CIRCUITSCAPE.
https://doi.org/10.1371/journal.pone.0111207.s017
Acknowledgments
We thank the Ministry of Environment and Forests, and the Chief Wildlife Wardens of Madhya Pradesh, Maharashtra, and Chhattisgarh states, India, for permissions and logistic support. We thank J. Borah, J. Vattakaven, K. Deka, Lalthanpuia, J. Jena, A. Bais, M. Aggarwal, G. George and the research fellows of the 2010 tiger assessment team for assisting with scat collection and laboratory work. The Director and Dean of WII are acknowledged for their support.
Author Contributions
Conceived and designed the experiments: YVJ QQ. Performed the experiments: BY YVJ QQ RG YS. Analyzed the data: BY YVJ QQ SS. Contributed reagents/materials/analysis tools: RG RCF QQ YVJ. Wrote the paper: BY YVJ. Field logistics: RG. Designed the genetic component of the study: BY. Scat sampling and laboratory analysis of genetic data: BY YVJ. Landscape scale occupancy data, field sample collection: YVJ QQ RG. Assisted in laboratory analysis genetic data: YS. Analyzed genetic data: BY. Supervision of genetic analysis: JEM RCF YVJ. Occupancy analysis: YVJ QQ. Corridor analysis: SS YVJ QQ. Critical revision of the manuscript: JEM RCF QQ.
- View Article
- Google Scholar
- 2. Gittleman JL, Funk SM, Macdonald DW, Wayne RK, eds (2001) Carnivore conservation . Cambridge: Cambridge University Press. 690 p.
- 18. Tilson RL, Seal US (1987) Tigers of the world. Noyes Publications, Park Ridge, New Jersey.
- 21. Sanderson E, Forrest J, Loucks C, Ginsberg J, Dinerstein E, et al. (2006) Setting Priorities for the Conservation and Recovery of Wild Tigers: 2005–2015. The Technical Assessment. Wildlife Conservation Society, World Wildlife Fund, Smithsonian Institution, and National Fish and Wildlife Foundation-Save the Tiger Fund, New York and Washington, D.C. 206 p.
- 24. Rangarajan M (1996) Fencing the forest: conservation and ecological change in India's Central Provinces, 1860–1914. Oxford University Press, New Delhi.245 p.
- 25. Rangarajan M (2006) India's wildlife history: an introduction. Permanent Black, New Delhi. 150 p.
- 26. Narain S, Panwar HS, Gadgil M, Thapar V (2005) Joining the dots: The report of the Tiger Task Force. Project Tiger, Union Ministry of Environment and Forests, New Delhi. Available: http://projecttiger.nic.in/WriteReadData/PublicationFile/full_report.pdf . Accessed 3 Jan 2013.
- 27. Anonymous (1972) The Indian Wildlife (Protection) Act, (1972). Ministry of Environment and Forests, Government of India. Nataraj Publishers, Dehradun, India.
- 28. Karanth KU, Gopal R (2005) An ecology-based policy framework for human-tiger coexistence in India. In: Woodroffe R, Thirgood S and Rabinowitz A, editors. People and Wildlife: Conflict or Coexistence? Cambridge University Press. pp.373–387.
- 30. Anonymous (2005) The Indian Wildlife (Protection) Act (1972) Amendment Act, 2005. Ministry of Environment and Forests, Government of India. Nataraj Publishers, Dehradun, India.
- 31. Jhala YV, Qureshi Q, Gopal R, Sinha PR, editors (2011) Status of the tigers, co-predators, and prey in India, 2010. National Tiger Conservation Authority, Ministry of Environment and Forests, Government of India, New Delhi and Wildlife Institute of India, Dehradun. TR2011/003. 302 p. Available: http://www.wii.gov.in/protected_download/publications/researchreports/2011/tiger/staus_tiger_2010 . Accessed 3 Mar 2013.
- 36. Hanski I, Gilpin M, eds (1997) Metapopulation biology: ecology, genetics and evolution. Academic Press, San Diego, California. 512 p.
- 37. Hanski I (1999) Metapopulation ecology. Oxford University Press, New York.313 p.
- 39. Seidensticker J, Dinerstein E, Goyal SP, Gurung B, Harihar A, et al. (2010) Tiger range collapse and recovery at the base of the Himalayas. In: Macdonald DW and Loveridge AJ, editors. Biology and Conservation of Wild Felids, Oxford University Press, Oxford, UK. pp.305–324.
- 40. Frankham R, Ballou JD, Briscoe D (2002) Introduction to Conservation Genetics. Cambridge University Press, Cambridge, UK.
- 42. Jhala YV, Gopal R, Qureshi Q, eds (2008) Status of the tigers, co-predators, and prey in India. National Tiger Conservation Authority, Ministry of Environment and Forests, Government of India, New Delhi and Wildlife Institute of India, Dehradun. TR08/001. 164 p. Available: http://www.wii.gov.in/protected_download/publications/statusof_tigers2008 . Accessed 13 Mar 2013.
- 43. Forest (Conservation) Act (1980) Handbook of Forest (Conservation) Act, 1980 (With Amendments made in 1988), Forest (Conservation) Rules, 2003 (With Amendments made in 2004), Guidelines & Clarifications (Up to June, 2004), Government of India, Ministry of Environment & Forest, New Delhi. 126 p. Available: http://www.arunachalpwd.org/pdf/Hanbook%20on%20Forest%20(Conservation)%20Act,%201980%20(updated%202004).pdf . Accessed 29 Mar 2013.
- 44. Fernandes A (2012) How coal mining is trashing tigerland. Greenpeace, Bengaluru, India. 115 p. Available: http://www.greenpeace.org/india/Global/india/report/How-Coal-mining-is-Trashing-Tigerland.pdf . Accessed 30 Mar 2013.
- 45. Pinjarkar V (2011) SC refuses to grant relief in NH7 four-laning along Pench. The Times of India, Nagpur.Available: http://articles.timesofindia.indiatimes.com/2011-04- 06/nagpur/29387911_1_laning-mansar-cuts-tiger-corridor. Accessed 28 Apr 2013.
- 46. Ramachandran R (2011) Ministry's ‘no’ to Neutrino Observatory project in Nilgiris. The Hindu. Available: http://www.thehindu.com/2009/11/21/stories/2009112154952000.htm . Accessed 23 Mar 2013.
- 47. Vattakaven J (2010) Fragmentation threat in Kanha-Pench corridor: Implications of the Gondia-Jabalpur railway line on corridor connectivity and tiger dispersal. WWF-India, New Delhi, 19 p. Available: http://awsassets.wwfindia.org/downloads/fragmentation_threat_in_the_kanha_pench_corridor_1.pdf . Accessed 15 Apr 2013.
- 67. Sunquist ME, Karanth KU, Sunquist F (1999) Ecology, behaviour and resilience of the tiger and its conservation needs. In: Seidensticker J, Christie S and Jackson P, editors. Riding the tiger: Tiger conservation in human dominated landscapes. Cambridge University Press, Cambridge, UK. pp.5–18.
- 70. MacKenzie DI, Nichols JD, Royles JA, Pollock KH, Bailey LL, et al. (2006) Occupancy estimation and modeling: inferring patterns and dynamics of species occurrence.Academic Press. 324 p.
- 72. McRae BH, Shah VB (2009) Circuitscape users' guide online. The University of California, Santa Barbara. Available: http://www.circuitscape.org . Accessed 10 Oct 2013.
- 87. Goudet J (2001) FSTAT, a program to estimate and test gene diversities and fixation indices (version 2.9.3). Available: http://www2.unil.ch/popgen/softwares/fstat.htm . Accessed 3 Jul 2012.
- 90. Savicky P (2009) pspearman: Spearman's rank correlation test. R package version 0.2–5. Available: http://CRAN.R-project.org/package=pspearman . Accessed 10 Jun 2014.
- 97. Beerli P (2008) Migrate-n, version 3.3.2: a maximum likelihood and Bayesian estimator of gene flow using the coalescent. Available: http://popgen.scs.fsu.edu/migrate-n.html . Accessed 4 May 2013.
- 107. Jhala YV, Qureshi Q, Gopal R, Amin R (2009) Field Guide: Monitoring tigers, co-predators, prey and their habitats. Third edition. Technical publication of National Tiger Conservation Authority, Government of India, New Delhi and Wildlife Institute of India, Dehradun, India. Available: http://www.wii.gov.in/images/field_guide_monitoring_tigers_co_predators.pdf . Accessed 28 Mar 2013.
- 108. Karanth KU, Nichols JD, eds (2002) Monitoring tigers and their prey. A manual for researchers, managers, and conservationists in Tropical Asia. Center for Wildlife Studies, Bangalore, India.193 p.
- 109. Forest Survey of India (2009) India: State of Forest Report. Ministry of Environment and Forests, Government of India, Dehradun, India. Available: http://fsi.nic.in/details.php?pgID=sb_61 . Accessed 15 March 2013.
- 110. Rodriguez E, Morris CS, Belz JE, Chapin EC, Martin JM, et al. (2005) An assessment of the SRTM topographic products, Jet Propulsion Laboratory Publications, D31639. 143 p.
- 114. Hines JE (2006) PRESENCE2 – Software to estimate patch occupancy and related parameters. Version 2.4. USGS-PWRC. Available: http://www.mbr-pwrc.usgs.gov/software/presence.html . Accessed 20 Feb 2013.
- 117. Jolliffe IT (2002) Principal Component Analysis. Springer, Heidelberg.493 p.
- 126. Pritchard J, Wen W, Falush D (2009) Documentation for STRUCTURE software: Version 2.3. Department of Human Genetics, University of Chicago, Chicago and Department of Statistics, University of Oxford, Oxford.
- 135. WWF India (2012) The return of tigers to Kopijhola, Madhya Pradesh, India: a tigress camera trapped in the Kopijhola forest block signifies its importance as a corridor. WWF–India Satpura Maikal Landscape Program. Available: http://www.wwfindia.org/news_facts/?uNewsID=7600 . Accessed 21 Oct 2013.
- 137. Beerli P (2009). How to use MIGRATE or why are Markov chain Monte Carlo programs so difficult to use. In: Bertorell G, Bruford MW, Hauffe HC, Rizzoli A, Vernesi C, editors. Population Genetics for Animal conservation. Cambridge University Press, Cambridge. pp.42–79.
- 144. Panwar HS (1987) Project Tiger: the reserves, the tigers and their future. In: Tilson RL and Seal US, editors. Tigers of the World: the biology, biopolitics, management and conservation of an endangered species. Noyes Publications, Park Ridge, New Jersey. pp.110–117.
- 148. Quintero J, Roca R, Morgan AJ, Mathur A (2010) Smart Green Infrastructure in Tiger Range Countries: A Multi-Level Approach. Global Tiger Initiative, GTI-SGI working group, technical paper. Accessed: http://www.globaltigerinitiative.org/download/GTI-Smart-Green-Infrastructure-Technical-Paper.pdf . Accessed 28 Jan 2013.
- 150. Pabla HS, Carlisle L, Cooper D, Cooke J, Nigam P, et al. (2011) Reintroduction of Gaur ( Bos gaurus gaurus ) in Bandhavgarh Tiger Reserve, India. Technical Report. Madhya Pradesh Forest Department, & Beyond and Wildlife Institute of India, Dehradun, India. 73p. Available: http://www.wii.gov.in/images/publications/researchreports/2011/reintroduction_gaur_report.pdf . Accessed 10 Sept 2013.
Information
- Author Services
Initiatives
You are accessing a machine-readable page. In order to be human-readable, please install an RSS reader.
All articles published by MDPI are made immediately available worldwide under an open access license. No special permission is required to reuse all or part of the article published by MDPI, including figures and tables. For articles published under an open access Creative Common CC BY license, any part of the article may be reused without permission provided that the original article is clearly cited. For more information, please refer to https://www.mdpi.com/openaccess .
Feature papers represent the most advanced research with significant potential for high impact in the field. A Feature Paper should be a substantial original Article that involves several techniques or approaches, provides an outlook for future research directions and describes possible research applications.
Feature papers are submitted upon individual invitation or recommendation by the scientific editors and must receive positive feedback from the reviewers.
Editor’s Choice articles are based on recommendations by the scientific editors of MDPI journals from around the world. Editors select a small number of articles recently published in the journal that they believe will be particularly interesting to readers, or important in the respective research area. The aim is to provide a snapshot of some of the most exciting work published in the various research areas of the journal.
Original Submission Date Received: .
- Active Journals
- Find a Journal
- Proceedings Series
- For Authors
- For Reviewers
- For Editors
- For Librarians
- For Publishers
- For Societies
- For Conference Organizers
- Open Access Policy
- Institutional Open Access Program
- Special Issues Guidelines
- Editorial Process
- Research and Publication Ethics
- Article Processing Charges
- Testimonials
- Preprints.org
- SciProfiles
- Encyclopedia
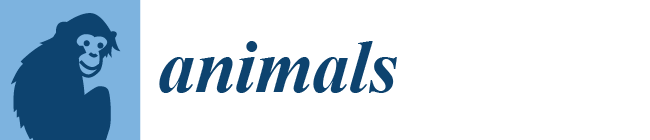
Article Menu

- Subscribe SciFeed
- Recommended Articles
- Google Scholar
- on Google Scholar
- Table of Contents
Find support for a specific problem in the support section of our website.
Please let us know what you think of our products and services.
Visit our dedicated information section to learn more about MDPI.
JSmol Viewer
Predicting the impact of climate change on the selection of reintroduction sites for the south china tiger ( panthera tigris amoyensis ) in china.
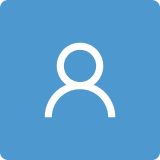
Simple Summary
1. introduction, 2. materials and methods, 2.1. data sources and processing, 2.1.1. prey species screening, 2.1.2. species distribution data search and screening, 2.1.3. environmental variable data sources and processing, 2.2. model optimization and construction, 2.3. drawing of distribution map of prey and habitat suitable for south china tiger, 2.4. discussion of the protection status of core alternative sites, 2.5. estimation of carrying capacity of core candidate sites, 3.1. changes in suitable habitats for prey under climate change, 3.2. distribution and changes of the reintroduction area of the south china tiger under climate change, 3.3. identify core candidate sites for priority protection, 3.4. core candidate sites can support the survival of small- and medium-sized populations of south china tigers, 4. discussion, 4.1. selection of prey, 4.2. impacts of climate change on prey and the south china tiger, 4.3. the reintroduction of the south china tiger into the wild requires a larger and more stable habitat, 4.4. assurance for reintroduction projects, 5. conclusions, supplementary materials, author contributions, institutional review board statement, informed consent statement, data availability statement, conflicts of interest.
- Mantyka-Pringle, C.S.; Visconti, P.; Di Marco, M.; Martin, T.G.; Rondinini, C.; Rhodes, J.R. Climate change modifies risk of global biodiversity loss due to land-cover change. Biol. Conserv. 2015 , 187 , 103–111. [ Google Scholar ] [ CrossRef ]
- Pimm, S.L. Biodiversity: Climate Change or Habitat Loss—Which Will Kill More Species? Curr. Biol. 2008 , 18 , R117–R119. [ Google Scholar ] [ CrossRef ]
- Koo, K.A.; Park, S.U.; Seo, C. Effects of Climate Change on the Climatic Niches of Warm-Adapted Evergreen Plants: Expansion or Contraction? Forests 2017 , 8 , 500. [ Google Scholar ] [ CrossRef ]
- Zhang, K.; Liu, Z.; Abdukeyum, N.; Ling, Y. Potential Geographical Distribution of Medicinal Plant Ephedra sinica Stapf under Climate Change. Forests 2022 , 13 , 2149. [ Google Scholar ] [ CrossRef ]
- Zhang, L.; Li, Q.; Kou, X.; Ouyang, Z. Distributions of two native ungulates at the third pole are highly sensitive to global warming. Glob. Ecol. Conserv. 2022 , 39 , e02292. [ Google Scholar ] [ CrossRef ]
- Ramasamy, M.; Das, B.; Ramesh, R. Predicting climate change impacts on potential worldwide distribution of fall armyworm based on CMIP6 projections. J. Pest Sci. 2022 , 95 , 841–854. [ Google Scholar ] [ CrossRef ]
- Tang, J.; Zhang, J.; Zhao, X.; Wei, W.; Hong, M.; Zhou, H.; Zhang, J.; Zhang, Z. The fate of giant panda and its sympatric mammals under future climate change. Biol. Conserv. 2022 , 274 , 109715. [ Google Scholar ] [ CrossRef ]
- Zhou, Y.; Zhang, Z.; Zhu, B.; Cheng, X.; Yang, L.; Gao, M.; Kong, R. MaxEnt Modeling Based on CMIP6 Models to Project Potential Suitable Zones for Cunninghamia lanceolata in China. Forests 2021 , 12 , 752. [ Google Scholar ] [ CrossRef ]
- Mao, C.; Ren, Q.; He, C.; Qi, T. Assessing direct and indirect impacts of human activities on natural habitats in the Qinghai-Tibet Plateau from 2000 to 2020. Ecol. Indic. 2023 , 157 , 111217. [ Google Scholar ] [ CrossRef ]
- Yang, L.; Xu, H.; Pan, S.; Chen, W.; Zeng, J. Identifying the impact of global human activities expansion on natural habitats. J. Clean. Prod. 2024 , 434 , 140247. [ Google Scholar ] [ CrossRef ]
- Correa Ayram, C.A.; Mendoza, M.E.; Etter, A.; Pérez Salicrup, D.R. Anthropogenic impact on habitat connectivity: A multidimensional human footprint index evaluated in a highly biodiverse landscape of Mexico. Ecol. Indic. 2017 , 72 , 895–909. [ Google Scholar ] [ CrossRef ]
- Roemer, G.W.; Gompper, M.E.; Van Valkenburgh, B. The Ecological Role of the Mammalian Mesocarnivore. BioScience 2009 , 59 , 165–173. [ Google Scholar ] [ CrossRef ]
- IUCN. The IUCN Red List of Threatened Species. Available online: https://www.iucnredlist.org/en (accessed on 29 February 2024).
- Tilson, R.; Defu, H.; Muntifering, J.; Nyhus, P.J. Dramatic decline of wild South China tigers Panthera tigris amoyensis : Field survey of priority tiger reserves. ORX 2004 , 38 , 40–47. [ Google Scholar ] [ CrossRef ]
- National Forestry and Grassland Administration (NFGA). Biodiversity Conservation in China. Available online: https://www.forestry.gov.cn/c/www/szxx/54502.jhtml (accessed on 25 February 2024).
- National Forestry and Grassland Administration (NFGA). Leaf Muntjac, Shoudai and Other Wild Animals Were Included in the New ‘Three Haves’ List of Wild Boar and So On. Available online: https://www.forestry.gov.cn/search/509801 (accessed on 2 December 2023).
- Karanth, K.U.; Stith, B.M. Prey depletion as a critical determinant of tiger population viability. In Riding the Tiger: Tiger Conservation in Humman-Dominated Landscapes ; Cambridge University Press: Cambridge, UK, 1999; pp. 100–113. [ Google Scholar ]
- Karanth, K.U.; Nichols, J.D.; Kumar, N.S.; Link, W.A.; Hines, J.E. Tigers and their prey: Predicting carnivore densities from prey abundance. Proc. Natl. Acad. Sci. USA 2004 , 101 , 4854–4858. [ Google Scholar ] [ CrossRef ]
- Karanth, K.U.; Sunquist, M.E. Prey selection by tiger, leopard and dhole in tropical forests. J. Anim. Ecol. 1995 , 64 , 439–450. [ Google Scholar ] [ CrossRef ]
- Karanth, K.U. Estimating tiger Panthera tigris populations from camera-trap data using capture—Recapture models. Biol. Conserv. 1995 , 71 , 333–338. [ Google Scholar ] [ CrossRef ]
- Sanderson, E.W.; Miquelle, D.G.; Fisher, K.; Harihar, A.; Clark, C.; Moy, J.; Potapov, P.; Robinson, N.; Royte, L.; Sampson, D.; et al. Range-wide trends in tiger conservation landscapes, 2001–2020. Front. Conserv. Sci. 2023 , 4 , 1191280. [ Google Scholar ] [ CrossRef ]
- Qin, Y.; Nyhus, P.J. Assessing factors influencing a possible South China tiger reintroduction: A survey of international conservation professionals. Environ. Conserv. 2018 , 45 , 58–66. [ Google Scholar ] [ CrossRef ]
- The Central People’s Goverment of the People’s Republic of China. WWF Positively Evaluated the ‘Chinese Wild Tiger Restoration Plan’. Available online: https://www.gov.cn/gzdt/2011-08/04/content_1919539.htm (accessed on 6 December 2023).
- The Central People’s Goverment of the People’s Republic of China. Forestry Bureau: China Is Preparing the “Chinese Wild Tiger Population Recovery Plan”. Available online: https://www.gov.cn/jrzg/2010-10/13/content_1722105.htm (accessed on 2 December 2023).
- Tilson, R.; Nyhus, P.J. Tigers of the World: The Science, Politics and Conservation of Panthera tigris , 2nd ed.; Academic Press: New York, NY, USA, 2010. [ Google Scholar ]
- Qin, Y.; Nyhus, P.J.; Larson, C.L.; Carroll, C.J.W.; Muntifering, J.; Dahmer, T.D.; Jun, L.; Tilson, R.L. An assessment of South China tiger reintroduction potential in Hupingshan and Houhe National Nature Reserves, China. Biol. Conserv. 2015 , 182 , 72–86. [ Google Scholar ] [ CrossRef ]
- Tang, T.; Li, J.; Sun, H.; Velázquez, J. Integrated approach considering seasonal variability and habitat uncertainty to map habitat for the prey of South China tiger. Ecol. Indic. 2023 , 148 , 110082. [ Google Scholar ] [ CrossRef ]
- Tang, T.; Li, J.; Sun, H.; Deng, C. Priority areas identified through spatial habitat suitability index and network analysis: Wild boar populations as proxies for tigers in and around the Hupingshan and Houhe National Nature Reserves. Sci. Total Environ. 2021 , 774 , 145067. [ Google Scholar ] [ CrossRef ]
- Wang, X.; He, J.; Mou, X.; Zhu, Z.; Chai, H.; Liu, G.; Rao, S.; Zhang, X. 20 Years of Ecological Protection and Restoration in China: Review and Prospect. Chin. J. Environ. Manag. 2021 , 13 , 85–92. [ Google Scholar ]
- Yuan, X.; Chen, W.; Lu, K.; Lu, Y.; Zhang, S. Investigation on South China Tigers and Their Habitats in Guangdong Province. Chin. J. Wildl. 1994 , 10–14. [ Google Scholar ] [ CrossRef ]
- Hayward, M.W.; Jędrzejewski, W.; Jêdrzejewska, B. Prey preference of the tiger Panthera tigris . J. Zool. 2012 , 286 , 221–231. [ Google Scholar ] [ CrossRef ]
- Tilson, R.; Traylor-Holzer, K.; Jiang, Q.M. The Decline and Impending Extinction of the South China Tiger ; Cambridge University Press: Cambridge, UK, 1997. [ Google Scholar ]
- Liang, J.; Ding, Z.; Jiang, Z.; Yang, X.; Xiao, R.; Singh, P.B.; Hu, Y.; Guo, K.; Zhang, Z.; Hu, H. Climate change, habitat connectivity, and conservation gaps: A case study of four ungulate species endemic to the Tibetan Plateau. Landsc. Ecol. 2021 , 36 , 1071–1087. [ Google Scholar ] [ CrossRef ]
- Malakoutikhah, S.; Fakheran, S.; Hemami, M.; Tarkesh, M.; Senn, J. Assessing future distribution, suitability of corridors and efficiency of protected areas to conserve vulnerable ungulates under climate change. Divers. Distrib. 2020 , 26 , 1383–1396. [ Google Scholar ] [ CrossRef ]
- Meng, B.; Huang, X.; Xie, B.; Wang, W.; Huang, J.; Zhang, T.; Ran, J.; Zhang, M. Distribution of suitable habitat for ungulates in Fanjingshan National Nature Reserve Reserve, Guizhou Province. Acta Theriol. Sin. 2023 , 43 , 664–675. [ Google Scholar ]
- Zhang, K.; Zhang, Y.; Tao, J. Predicting the Potential Distribution of Paeonia veitchii (Paeoniaceae) in China by Incorporating Climate Change into a Maxent Model. Forests 2019 , 10 , 190. [ Google Scholar ] [ CrossRef ]
- Phillips, S.J.; Anderson, R.P.; Schapire, R.E. Maximum entropy modeling of species geographic distributions. Ecol. Model. 2006 , 190 , 231–259. [ Google Scholar ] [ CrossRef ]
- Cobos, M.E.; Peterson, A.T.; Barve, N.; Osorio-Olvera, L. kuenm: An R package for detailed development of ecological niche models using Maxent. PeerJ 2019 , 7 , e6281. [ Google Scholar ] [ CrossRef ]
- Muscarella, R.; Galante, P.J.; Soley-Guardia, M.; Boria, R.A.; Kass, J.M.; Uriarte, M.; Anderson, R.P. ENM eval: An R package for conducting spatially independent evaluations and estimating optimal model complexity for Maxent ecological niche models. Methods Ecol. Evol. 2014 , 5 , 1198–1205. [ Google Scholar ] [ CrossRef ]
- Fielding, A.H.; Bell, J.F. A review of methods for the assessment of prediction errors in conservation presence/absence models. Environ. Conserv. 1997 , 24 , 38–49. [ Google Scholar ] [ CrossRef ]
- Shcheglovitova, M.; Anderson, R.P. Estimating optimal complexity for ecological niche models: A jackknife approach for species with small sample sizes. Ecol. Model. 2013 , 269 , 9–17. [ Google Scholar ] [ CrossRef ]
- Swets, J.A. Measuring the Accuracy of Diagnostic Systems. Science 1988 , 240 , 1285–1293. [ Google Scholar ] [ CrossRef ]
- Mu, H.; Li, X.; Wen, Y.; Huang, J.; Du, P.; Su, W.; Miao, S.; Geng, M. A global record of annual terrestrial Human Footprint dataset from 2000 to 2018. Sci. Data 2022 , 9 , 176. [ Google Scholar ] [ CrossRef ]
- O’Brien, T.G.; Kinnaird, M.F.; Wibisono, H.T. Crouching tigers, hidden prey: Sumatran tiger and prey populations in a tropical forest landscape. Anim. Conserv. 2003 , 6 , 131–139. [ Google Scholar ] [ CrossRef ]
- Sunquist, M. Chapter2-What Is a Tiger? Ecology and Behavior. In Tigers of the World: The Science, Politics and Conservation of Panthera tigris , 2nd ed.; William Andrew Publishing: Norwich, CT, USA, 2010; pp. 19–33. [ Google Scholar ]
- Zeng, J.; Hu, J.; Shi, Y.; Li, Y.; Guo, Z.; Wang, S.; Song, S. Effects of Climate Change on the Habitat of the Leopard ( Panthera pardus ) in the Liupanshan National Nature Reserve of China. Animals 2022 , 12 , 1866. [ Google Scholar ] [ CrossRef ]
- Li, W.; Shi, M.; Huang, Y.; Chen, K.; Sun, H.; Chen, J. Climatic Change Can Influence Species Diversity Patterns and Potential Habitats of Salicaceae Plants in China. Forests 2019 , 10 , 220. [ Google Scholar ] [ CrossRef ]
- Markov, N.; Pankova, N.; Morelle, K. Where winter rules: Modeling wild boar distribution in its north-eastern range. Sci. Total Environ. 2019 , 687 , 1055–1064. [ Google Scholar ] [ CrossRef ]
- Awasthi, N.; Kumar, U.; Qureshi, Q.; Pradhan, A.; Chauhan, J.S.; Jhala, Y.V. Effect of human use, season and habitat on ungulate density in Kanha Tiger Reserve, Madhya Pradesh, India. Reg. Environ. Change 2016 , 16 , 31–41. [ Google Scholar ] [ CrossRef ]
- Biswas, S.; Kumar, S.; Bandhopadhyay, M.; Patel, S.K.; Lyngdoh, S.; Pandav, B.; Mondol, S. What drives prey selection? Assessment of Tiger ( Panthera tigris ) food habits across the Terai-Arc Landscape, India. MAMM 2023 , 104 , 1302–1316. [ Google Scholar ] [ CrossRef ]
- Dahal, B.R.; Amin, R.; Lamichhane, B.R.; Giri, S.R.; Acharya, H.; Acharya, H.R.; Harihar, A. Setting recovery targets for a charismatic species in an iconic protected area complex: The case of tigers ( Panthera tigris ) in Chitwan–Parsa National Parks, Nepal. Conserv. Sci. Pract. 2023 , 5 , e12930. [ Google Scholar ] [ CrossRef ]
- Qi, J.; Gu, J.; Ning, Y.; Miquelle, D.G.; Holyoak, M.; Wen, D.; Liang, X.; Liu, S.; Roberts, N.J.; Yang, E.; et al. Integrated assessments call for establishing a sustainable meta-population of Amur tigers in northeast Asia. Biol. Conserv. 2021 , 261 , 109250. [ Google Scholar ] [ CrossRef ]
- Mehlich, R. A Preliminary Habitat Suitability Analysis for the Restoration of South China Tigers in the Hupingshan Reserve, China. Atlas Maine 2005 , 2005 , 13. [ Google Scholar ]
- Letro, L.; Fischer, K.; Duba, D.; Tandin, T. Occupancy patterns of prey species in a biological corridor and inferences for tiger population connectivity between national parks in Bhutan. Oryx 2022 , 56 , 421–428. [ Google Scholar ] [ CrossRef ]
- Penjor, U.; Kaszta, Ż.; Macdonald, D.W.; Cushman, S.A. Prioritizing areas for conservation outside the existing protected area network in Bhutan: The use of multi-species, multi-scale habitat suitability models. Landsc. Ecol. 2021 , 36 , 1281–1309. [ Google Scholar ] [ CrossRef ]
- Thinley, P.; Rajaratnam, R.; Morreale, S.J.; Lassoie, J.P. Assessing the adequacy of a protected area network in conserving a wide-ranging apex predator: The case for tiger ( Panthera tigris ) conservation in Bhutan. Conserv. Sci. Pract. 2021 , 3 , e318. [ Google Scholar ] [ CrossRef ]
- Gray, T.N.E.; Crouthers, R.; Ramesh, K.; Vattakaven, J.; Borah, J.; Pasha, M.K.S.; Lim, T.; Phan, C.; Singh, R.; Long, B.; et al. A framework for assessing readiness for tiger Panthera tigris reintroduction: A case study from eastern Cambodia. Biodivers. Conserv. 2017 , 26 , 2383–2399. [ Google Scholar ] [ CrossRef ]
- Jhala, Y.; Gopal, R.; Mathur, V.; Ghosh, P.; Negi, H.S.; Narain, S.; Yadav, S.P.; Malik, A.; Garawad, R.; Qureshi, Q. Recovery of tigers in India: Critical introspection and potential lessons. People Nat. 2021 , 3 , 281–293. [ Google Scholar ] [ CrossRef ]
- Belote, R.T.; Wilson, M.B. Delineating greater ecosystems around protected areas to guide conservation. Conserv. Sci. Pract. 2020 , 2 , e196. [ Google Scholar ] [ CrossRef ]
- Dinerstein, E.; Joshi, A.R.; Vynne, C.; Lee, A.T.L.; Pharand-Deschênes, F.; França, M.; Fernando, S.; Birch, T.; Burkart, K.; Asner, G.P.; et al. A “Global Safety Net” to reverse biodiversity loss and stabilize earth’s climate. Sci. Adv. 2020 , 6 , eabb2824. [ Google Scholar ] [ CrossRef ] [ PubMed ]
- Dinerstein, E.; Loucks, C.; Wikramanayake, E.; Ginsberg, J.; Sanderson, E.; Seidensticker, J.; Forrest, J.; Bryja, G.; Heydlauff, A.; Klenzendorf, S.; et al. The Fate of Wild Tigers. BioScience 2007 , 57 , 508–514. [ Google Scholar ] [ CrossRef ]
- Kenney, J.S.; Smith, J.L.D.; Starfield, A.M.; Mcdougal, C.W. The Long-Term Effects of Tiger Poaching on Population Viability. Conserv. Biol. 1995 , 9 , 1127–1133. [ Google Scholar ] [ CrossRef ] [ PubMed ]
- Linkie, M.; Chapron, G.; Martyr, D.J.; Holden, J.; Leader-Williams, N. Assessing the viability of tiger subpopulations in a fragmented landscape. J. Appl. Ecol. 2006 , 43 , 576–586. [ Google Scholar ] [ CrossRef ]
- Carter, N.; Killion, A.; Easter, T.; Brandt, J.; Ford, A. Road development in Asia: Assessing the range-wide risks to tigers. Sci. Adv. 2020 , 6 , eaaz9619. [ Google Scholar ] [ CrossRef ]
- Dertien, J.S.; Negi, H.; Dinerstein, E.; Krishnamurthy, R.; Negi, H.S.; Gopal, R.; Gulick, S.; Pathak, S.K.; Kapoor, M.; Yadav, P.; et al. Mitigating human-wildlife conflict and monitoring endangered tigers using a real-time camera-based alert system. BioScience 2023 , 73 , 748–757. [ Google Scholar ] [ CrossRef ]
- Rathore, C.S.; Dubey, Y.; Shrivastava, A.; Pathak, P.; Patil, V. Opportunities of Habitat Connectivity for Tiger ( Panthera tigris ) between Kanha and Pench National Parks in Madhya Pradesh, India. PLoS ONE 2012 , 7 , e39996. [ Google Scholar ] [ CrossRef ]
- Wen, D.; Qi, J.; Long, Z.; Gu, J.; Tian, Y.; Roberts, N.J.; Yang, E.; Kong, W.; Zhao, Y.; Sun, Q.; et al. Conservation potentials and limitations of large carnivores in protected areas: A case study in Northeast China. Conserv. Sci. Pract. 2022 , 4 , e12693. [ Google Scholar ] [ CrossRef ]
- Fàbregas, M.C.; Fosgate, G.T.; Koehler, G.M. Hunting performance of captive-born South China tigers ( Panthera tigris amoyensis ) on free-ranging prey and implications for their reintroduction. Biol. Conserv. 2015 , 192 , 57–64. [ Google Scholar ] [ CrossRef ]
- Wang, C.; Wu, D.-D.; Yuan, Y.-H.; Yao, M.-C.; Han, J.-L.; Wu, Y.-J.; Shan, F.; Li, W.-P.; Zhai, J.-Q.; Huang, M.; et al. Population genomic analysis provides evidence of the past success and future potential of South China tiger captive conservation. BMC Biol. 2023 , 21 , 64. [ Google Scholar ] [ CrossRef ]
- Ning, Y.; Liu, D.; Gu, J.; Zhang, Y.; Roberts, N.J.; Guskov, V.Y.; Sun, J.; Liu, D.; Gong, M.; Qi, J.; et al. The genetic status and rescue measure for a geographically isolated population of Amur tigers. Sci. Rep. 2024 , 14 , 8088. [ Google Scholar ] [ CrossRef ] [ PubMed ]
- Sarkar, M.S.; Ramesh, K.; Johnson, J.A.; Sen, S.; Nigam, P.; Gupta, S.K.; Murthy, R.S.; Saha, G.K. Movement and home range characteristics of reintroduced tiger ( Panthera tigris ) population in Panna Tiger Reserve, central India. Eur. J. Wildl. Res. 2016 , 62 , 537–547. [ Google Scholar ] [ CrossRef ]
- Chundawat, R.S.; Sharma, K.; Gogate, N.; Malik, P.K.; Vanak, A.T. Size matters: Scale mismatch between space use patterns of tigers and protected area size in a Tropical Dry Forest. Biol. Conserv. 2016 , 197 , 146–153. [ Google Scholar ] [ CrossRef ]
- Dendup, P.; Lham, C.; Wangchuk, W.; Jamtsho, Y. Tiger abundance and ecology in Jigme Dorji National Park, Bhutan. Glob. Ecol. Conserv. 2023 , 42 , e02378. [ Google Scholar ] [ CrossRef ]
- Duangchantrasiri, S.; Umponjan, M.; Simcharoen, S.; Pattanavibool, A.; Chaiwattana, S.; Maneerat, S.; Kumar, N.S.; Jathanna, D.; Srivathsa, A.; Karanth, K.U. Dynamics of a low-density tiger population in Southeast Asia in the context of improved law enforcement. Conserv. Biol. 2016 , 30 , 639–648. [ Google Scholar ] [ CrossRef ]
- Phumanee, W.; Steinmetz, R.; Phoonjampa, R.; Weingdow, S.; Phokamanee, S.; Bhumpakphan, N.; Savini, T. Tiger density, movements, and immigration outside of a tiger source site in Thailand. Conserv. Sci. Pract. 2021 , 3 , e560. [ Google Scholar ] [ CrossRef ]
- Sarkar, M.S.; Niyogi, R.; Masih, R.L.; Hazra, P.; Maiorano, L.; John, R. Long-distance dispersal and home range establishment by a female sub-adult tiger ( Panthera tigris ) in the Panna landscape, central India. Eur. J. Wildl. Res. 2021 , 67 , 54. [ Google Scholar ] [ CrossRef ]
Click here to enlarge figure
Family | Genus | Species | English Name | Weight Range (kg) | National Protection Level | Number of Distribution Points |
---|---|---|---|---|---|---|
Bovidae | Capricornis | Capricornis milneedwardsi | Chinese serow | 85–140 | II | 53 |
Naemorhedus | Naemorhedus griseus | Chinese goral | 40–50 | II | 36 | |
Cervidae | Elaphodus | Elaphodus cephalophus | Tufted deer | 15–28 | II | 95 |
Hydropotes | Hydropotes inermis | Water deer | 15–20 | II | 20 | |
Muntiacus | Muntiacus reevesi | Chinese muntjac | 9–18 | — | 69 | |
Muntiacus vaginalis | Red muntjac | 20–33 | — | 102 | ||
Rusa | Rusa unicolor | Sambar | 100–200 | II | 26 | |
Suidae | Sus | Sus scrofa | Wild boar | 90–200 | — | 98 |
Category | Code | Environmental Factors | Resolution | Data Sources |
---|---|---|---|---|
Climatic factors | bio01 | Annual mean temperature | 30 s | WorldClim ( , accessed on 12 July 2023) |
bio02 | Mean diural range | |||
bio03 | Isothermality | |||
bio04 | Temperature seasonality | |||
bio05 | Max temperature of warmest month | |||
bio06 | Min temperature of coldest month | |||
bio07 | Temperature annual range | |||
bio08 | Mean temperature of wettest quarter | |||
bio09 | Mean temperature of driest quarter | |||
bio10 | Mean temperature of warmest quarter | |||
bio11 | Mean temperature of coldest quarter | |||
bio12 | Annual precipitation | |||
bio13 | Precipitation of wettest month | |||
bio14 | Precipitation of driest month | |||
bio15 | Precipitation seasonality | |||
bio16 | Precipitation of wettest quarter | |||
bio17 | Precipitation of driest quarter | |||
bio18 | Precipitation of warmest quarter | |||
bio19 | Precipitation of coldest quarter | |||
Terrain factors | altitude | Altitude | 90 m | Geospatial Data Cloud ( , accessed on 26 July 2023) |
slope | Slope | |||
aspect | Aspect | |||
Water source and vegetation factors | ndvi | Normalized Dierential Vegetation Index | 1 km | Resource and Environment Science and Data Center ( , accessed on 26 July 2023) |
veg | Vegetation type | |||
dis_river | Distance from water sources | 90 m | Geospatial Data Cloud ( , accessed on 26 July 2023) | |
Human disturbance factors | dis_human | Distance from residential areas | 1 km | National Catalogue Service For Geographic Information ( , accessed on 3 August 2023) |
dis_road | Distance from road | / | open street map ( , accessed on 3 August 2023) |
Species | Habitat Suitability | Habitat Area Now (km ) | Habitat Area in 2050 (km ) | Habitat Area in 2070 (km ) | Habitat Change by 2050 (%) | Habitat Change by 2070 (%) |
---|---|---|---|---|---|---|
Capricornis milneedwardsi | High suitable | 54,876 | 50,138 | 27,868 | −8.63% | −49.21% |
Medium suitable | 170,466 | 179,324 | 174,466 | 5.19% | 2.34% | |
Low suitable | 467,156 | 568,799 | 526,289 | 21.75% | 12.65% | |
Naemorhedus griseus | High suitable | 369,149 | 170,345 | 92,282 | −53.85% | −75.00% |
Medium suitable | 640,326 | 493,868 | 397,301 | −22.87% | −37.95% | |
Low suitable | 1,834,674 | 1,400,069 | 1,167,314 | −23.68% | −36.37% | |
Elaphodus cephalophus | High suitable | 213,434 | 236,695 | 76,661 | 10.89% | −64.08% |
Medium suitable | 372,400 | 366,222 | 306,692 | −1.65% | −17.64% | |
Low suitable | 735,084 | 738,912 | 676,538 | 0.52% | −7.96% | |
Hydropotes inermis | High suitable | 1,004,282 | 958,594 | 435,657 | −4.54% | −56.62% |
Medium suitable | 647,448 | 789,096 | 1,158,562 | 21.87% | 78.94% | |
Low suitable | 2,014,916 | 2,081,083 | 1,850,296 | 3.28% | −8.17% | |
Muntiacus reevesi | High suitable | 82,783 | 11,159 | 5184 | −86.52% | −93.73% |
Medium suitable | 249,809 | 58,842 | 29,950 | −76.45% | −88.01% | |
Low suitable | 606,564 | 330,524 | 241,320 | −45.51% | −60.21% | |
Muntiacus vaginalis | High suitable | 45,199 | 132,459 | 120,919 | 193.05% | 167.52% |
Medium suitable | 131,419 | 208,282 | 189,466 | 58.48% | 44.16% | |
Low suitable | 479,220 | 443,652 | 392,290 | −7.42% | −18.13% | |
Rusa unicolor | High suitable | 414,874 | 694,872 | 770,772 | 67.48% | 85.78% |
Medium suitable | 1,074,348 | 1,518,966 | 1,760,765 | 41.38% | 63.89% | |
Low suitable | 1,969,101 | 2,364,065 | 2,512,421 | 20.05% | 27.59% | |
Sus scrofa | High suitable | 645,192 | 609,309 | 522,649 | −5.56% | −18.99% |
Medium suitable | 1,665,352 | 1,598,482 | 1,439,938 | −4.01% | −13.53% | |
Low suitable | 1,715,613 | 2,010,754 | 2,047,950 | 17.20% | 19.37% | |
Panthera tigris amoyensis | High suitable | 240,184 | 169,345 | 85,496 | −29.49% | −64.40% |
Medium suitable | 962,382 | 904,320 | 716,603 | −6.03% | −25.53% | |
Low suitable | 2,176,002 | 2,665,639 | 2,829,683 | 22.50% | 30.04% |
Patch Number | Patch Area/km | Protected Areas Involved | Number of Tigers |
---|---|---|---|
1 | 10,054.10 | Cibagou NNR | 23 M, 66 F |
2 | 7518.06 | Giant Panda NP, Heizhugou NNR, Mabian Dafengding NNR, Meigu Dafengding NNR, Bayuelin NR, Mamize NR, Maanshan NR | 17 M, 49 F |
3 | 7002.50 | Giant Panda NP | 16 M, 46 F |
4 | 3667.27 | / | 8 M, 23 F |
5 | 3608.42 | / | 8 M, 23 F |
6 | 2769.58 | Wuyishan NP, Tongbo mountain NR | 6 M, 18 F |
7 | 2736.27 | Giant Panda NP, Jiuzhaigou NR | 6 M, 18 F |
8 | 2619.86 | Jiulongshan NNR | 5 M, 17 F |
9 | 2311.22 | Baima Snow Mountain NNR | 5 M, 15 F |
10 | 1995.02 | Huaping NNR, Maoershan NNR, Nanshan NP | 4 M, 13 F |
11 | 1932.74 | Giant Panda NP | 4 M, 12 F |
12 | 1433.13 | Bamian Mountain NNR, Taoyuandong NNR, Jinggangshan NNR | 3 M, 9 F |
13 | 1234.82 | / | 2 M, 8 F |
14 | 1091.61 | Gaoligong Mountain NNR | 2 M, 7 F |
15 | 1078.57 | Meihuashan NNR | 2 M, 7 F |
16 | 1021.09 | / | 2 M, 6 F |
17 | 1018.29 | Yarlung Zangbo Grand Canyon NNR | 2 M, 6 F |
18 | 991.45 | Hubeishan NNR, Houhe NNR, Mulinzi NNR | 2 M, 6 F |
19 | 925.61 | Nanling NNR, Mangshan NNR | 2 M, 6 F |
20 | 793.57 | Qizimei Mountain NNR, Badagongshan NNR | 1 M, 5 F |
The statements, opinions and data contained in all publications are solely those of the individual author(s) and contributor(s) and not of MDPI and/or the editor(s). MDPI and/or the editor(s) disclaim responsibility for any injury to people or property resulting from any ideas, methods, instructions or products referred to in the content. |
Share and Cite
Luo, Y.; Xu, J.; Zhang, X.; Hou, Y. Predicting the Impact of Climate Change on the Selection of Reintroduction Sites for the South China Tiger ( Panthera tigris amoyensis ) in China. Animals 2024 , 14 , 2477. https://doi.org/10.3390/ani14172477
Luo Y, Xu J, Zhang X, Hou Y. Predicting the Impact of Climate Change on the Selection of Reintroduction Sites for the South China Tiger ( Panthera tigris amoyensis ) in China. Animals . 2024; 14(17):2477. https://doi.org/10.3390/ani14172477
Luo, Yueqing, Jin Xu, Xinyi Zhang, and Yulin Hou. 2024. "Predicting the Impact of Climate Change on the Selection of Reintroduction Sites for the South China Tiger ( Panthera tigris amoyensis ) in China" Animals 14, no. 17: 2477. https://doi.org/10.3390/ani14172477
Article Metrics
Article access statistics, supplementary material.
ZIP-Document (ZIP, 951 KiB)
Further Information
Mdpi initiatives, follow mdpi.
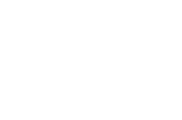
Subscribe to receive issue release notifications and newsletters from MDPI journals

Samantha Putterman, PolitiFact Samantha Putterman, PolitiFact
Leave your feedback
- Copy URL https://www.pbs.org/newshour/politics/fact-checking-warnings-from-democrats-about-project-2025-and-donald-trump
Fact-checking warnings from Democrats about Project 2025 and Donald Trump
This fact check originally appeared on PolitiFact .
Project 2025 has a starring role in this week’s Democratic National Convention.
And it was front and center on Night 1.
WATCH: Hauling large copy of Project 2025, Michigan state Sen. McMorrow speaks at 2024 DNC
“This is Project 2025,” Michigan state Sen. Mallory McMorrow, D-Royal Oak, said as she laid a hardbound copy of the 900-page document on the lectern. “Over the next four nights, you are going to hear a lot about what is in this 900-page document. Why? Because this is the Republican blueprint for a second Trump term.”
Vice President Kamala Harris, the Democratic presidential nominee, has warned Americans about “Trump’s Project 2025” agenda — even though former President Donald Trump doesn’t claim the conservative presidential transition document.
“Donald Trump wants to take our country backward,” Harris said July 23 in Milwaukee. “He and his extreme Project 2025 agenda will weaken the middle class. Like, we know we got to take this seriously, and can you believe they put that thing in writing?”
Minnesota Gov. Tim Walz, Harris’ running mate, has joined in on the talking point.
“Don’t believe (Trump) when he’s playing dumb about this Project 2025. He knows exactly what it’ll do,” Walz said Aug. 9 in Glendale, Arizona.
Trump’s campaign has worked to build distance from the project, which the Heritage Foundation, a conservative think tank, led with contributions from dozens of conservative groups.
Much of the plan calls for extensive executive-branch overhauls and draws on both long-standing conservative principles, such as tax cuts, and more recent culture war issues. It lays out recommendations for disbanding the Commerce and Education departments, eliminating certain climate protections and consolidating more power to the president.
Project 2025 offers a sweeping vision for a Republican-led executive branch, and some of its policies mirror Trump’s 2024 agenda, But Harris and her presidential campaign have at times gone too far in describing what the project calls for and how closely the plans overlap with Trump’s campaign.
PolitiFact researched Harris’ warnings about how the plan would affect reproductive rights, federal entitlement programs and education, just as we did for President Joe Biden’s Project 2025 rhetoric. Here’s what the project does and doesn’t call for, and how it squares with Trump’s positions.
Are Trump and Project 2025 connected?
To distance himself from Project 2025 amid the Democratic attacks, Trump wrote on Truth Social that he “knows nothing” about it and has “no idea” who is in charge of it. (CNN identified at least 140 former advisers from the Trump administration who have been involved.)
The Heritage Foundation sought contributions from more than 100 conservative organizations for its policy vision for the next Republican presidency, which was published in 2023.
Project 2025 is now winding down some of its policy operations, and director Paul Dans, a former Trump administration official, is stepping down, The Washington Post reported July 30. Trump campaign managers Susie Wiles and Chris LaCivita denounced the document.
WATCH: A look at the Project 2025 plan to reshape government and Trump’s links to its authors
However, Project 2025 contributors include a number of high-ranking officials from Trump’s first administration, including former White House adviser Peter Navarro and former Housing and Urban Development Secretary Ben Carson.
A recently released recording of Russell Vought, a Project 2025 author and the former director of Trump’s Office of Management and Budget, showed Vought saying Trump’s “very supportive of what we do.” He said Trump was only distancing himself because Democrats were making a bogeyman out of the document.
Project 2025 wouldn’t ban abortion outright, but would curtail access
The Harris campaign shared a graphic on X that claimed “Trump’s Project 2025 plan for workers” would “go after birth control and ban abortion nationwide.”
The plan doesn’t call to ban abortion nationwide, though its recommendations could curtail some contraceptives and limit abortion access.
What’s known about Trump’s abortion agenda neither lines up with Harris’ description nor Project 2025’s wish list.
Project 2025 says the Department of Health and Human Services Department should “return to being known as the Department of Life by explicitly rejecting the notion that abortion is health care.”
It recommends that the Food and Drug Administration reverse its 2000 approval of mifepristone, the first pill taken in a two-drug regimen for a medication abortion. Medication is the most common form of abortion in the U.S. — accounting for around 63 percent in 2023.
If mifepristone were to remain approved, Project 2025 recommends new rules, such as cutting its use from 10 weeks into pregnancy to seven. It would have to be provided to patients in person — part of the group’s efforts to limit access to the drug by mail. In June, the U.S. Supreme Court rejected a legal challenge to mifepristone’s FDA approval over procedural grounds.
WATCH: Trump’s plans for health care and reproductive rights if he returns to White House The manual also calls for the Justice Department to enforce the 1873 Comstock Act on mifepristone, which bans the mailing of “obscene” materials. Abortion access supporters fear that a strict interpretation of the law could go further to ban mailing the materials used in procedural abortions, such as surgical instruments and equipment.
The plan proposes withholding federal money from states that don’t report to the Centers for Disease Control and Prevention how many abortions take place within their borders. The plan also would prohibit abortion providers, such as Planned Parenthood, from receiving Medicaid funds. It also calls for the Department of Health and Human Services to ensure that the training of medical professionals, including doctors and nurses, omits abortion training.
The document says some forms of emergency contraception — particularly Ella, a pill that can be taken within five days of unprotected sex to prevent pregnancy — should be excluded from no-cost coverage. The Affordable Care Act requires most private health insurers to cover recommended preventive services, which involves a range of birth control methods, including emergency contraception.
Trump has recently said states should decide abortion regulations and that he wouldn’t block access to contraceptives. Trump said during his June 27 debate with Biden that he wouldn’t ban mifepristone after the Supreme Court “approved” it. But the court rejected the lawsuit based on standing, not the case’s merits. He has not weighed in on the Comstock Act or said whether he supports it being used to block abortion medication, or other kinds of abortions.
Project 2025 doesn’t call for cutting Social Security, but proposes some changes to Medicare
“When you read (Project 2025),” Harris told a crowd July 23 in Wisconsin, “you will see, Donald Trump intends to cut Social Security and Medicare.”
The Project 2025 document does not call for Social Security cuts. None of its 10 references to Social Security addresses plans for cutting the program.
Harris also misleads about Trump’s Social Security views.
In his earlier campaigns and before he was a politician, Trump said about a half-dozen times that he’s open to major overhauls of Social Security, including cuts and privatization. More recently, in a March 2024 CNBC interview, Trump said of entitlement programs such as Social Security, “There’s a lot you can do in terms of entitlements, in terms of cutting.” However, he quickly walked that statement back, and his CNBC comment stands at odds with essentially everything else Trump has said during the 2024 presidential campaign.
Trump’s campaign website says that not “a single penny” should be cut from Social Security. We rated Harris’ claim that Trump intends to cut Social Security Mostly False.
Project 2025 does propose changes to Medicare, including making Medicare Advantage, the private insurance offering in Medicare, the “default” enrollment option. Unlike Original Medicare, Medicare Advantage plans have provider networks and can also require prior authorization, meaning that the plan can approve or deny certain services. Original Medicare plans don’t have prior authorization requirements.
The manual also calls for repealing health policies enacted under Biden, such as the Inflation Reduction Act. The law enabled Medicare to negotiate with drugmakers for the first time in history, and recently resulted in an agreement with drug companies to lower the prices of 10 expensive prescriptions for Medicare enrollees.
Trump, however, has said repeatedly during the 2024 presidential campaign that he will not cut Medicare.
Project 2025 would eliminate the Education Department, which Trump supports
The Harris campaign said Project 2025 would “eliminate the U.S. Department of Education” — and that’s accurate. Project 2025 says federal education policy “should be limited and, ultimately, the federal Department of Education should be eliminated.” The plan scales back the federal government’s role in education policy and devolves the functions that remain to other agencies.
Aside from eliminating the department, the project also proposes scrapping the Biden administration’s Title IX revision, which prohibits discrimination based on sexual orientation and gender identity. It also would let states opt out of federal education programs and calls for passing a federal parents’ bill of rights similar to ones passed in some Republican-led state legislatures.
Republicans, including Trump, have pledged to close the department, which gained its status in 1979 within Democratic President Jimmy Carter’s presidential Cabinet.
In one of his Agenda 47 policy videos, Trump promised to close the department and “to send all education work and needs back to the states.” Eliminating the department would have to go through Congress.
What Project 2025, Trump would do on overtime pay
In the graphic, the Harris campaign says Project 2025 allows “employers to stop paying workers for overtime work.”
The plan doesn’t call for banning overtime wages. It recommends changes to some Occupational Safety and Health Administration, or OSHA, regulations and to overtime rules. Some changes, if enacted, could result in some people losing overtime protections, experts told us.
The document proposes that the Labor Department maintain an overtime threshold “that does not punish businesses in lower-cost regions (e.g., the southeast United States).” This threshold is the amount of money executive, administrative or professional employees need to make for an employer to exempt them from overtime pay under the Fair Labor Standards Act.
In 2019, the Trump’s administration finalized a rule that expanded overtime pay eligibility to most salaried workers earning less than about $35,568, which it said made about 1.3 million more workers eligible for overtime pay. The Trump-era threshold is high enough to cover most line workers in lower-cost regions, Project 2025 said.
The Biden administration raised that threshold to $43,888 beginning July 1, and that will rise to $58,656 on Jan. 1, 2025. That would grant overtime eligibility to about 4 million workers, the Labor Department said.
It’s unclear how many workers Project 2025’s proposal to return to the Trump-era overtime threshold in some parts of the country would affect, but experts said some would presumably lose the right to overtime wages.
Other overtime proposals in Project 2025’s plan include allowing some workers to choose to accumulate paid time off instead of overtime pay, or to work more hours in one week and fewer in the next, rather than receive overtime.
Trump’s past with overtime pay is complicated. In 2016, the Obama administration said it would raise the overtime to salaried workers earning less than $47,476 a year, about double the exemption level set in 2004 of $23,660 a year.
But when a judge blocked the Obama rule, the Trump administration didn’t challenge the court ruling. Instead it set its own overtime threshold, which raised the amount, but by less than Obama.
Support Provided By: Learn more
Educate your inbox
Subscribe to Here’s the Deal, our politics newsletter for analysis you won’t find anywhere else.
Thank you. Please check your inbox to confirm.

Research in 60 Seconds: Using Tech to Improve Readability
Associate Professor Ben Sawyer’s research examines how digital enhancements to text can help improve reading comprehension and speed.
By Nicole Dudenhoefer ’17 | Video by UCF Social | August 30, 2024
Whether it’s solving the world’s biggest problems or investigating the potential of novel discoveries, researchers at UCF are on the edge scientific breakthroughs that aim to make an impact. Through the Research in 60 Seconds series , student and faculty researchers condense their complex studies into bite-sized summaries so you can know how and why Knights plan to improve our world.
Name: Ben Sawyer
Position(s): Associate professor of industrial engineering and director of The Readability Consortium
Why are you interested in this research? My mother was a children’s librarian focused on building collections, and my father was a high school teacher, and then a professor of education. My own early work was focused on attention and distraction, and I became fascinated by how people get information out of machine systems, and into their minds. My present research centers around human performance in reading: how can we best move information into your awareness, so you can do something with it.
Are you a faculty member or student conducting research at UCF? We want to hear from you! Tell us about your research at bit.ly/ucf-research-60-form.
Who inspires you to conduct your research? I’m inspired by people working hard to understand [information, including] children, soldiers, analysts, physicians, and older adults all looking to find the information they need to get them to their goal and keep them safe on the way. My father worked with children with dyslexia, and it’s amazing how much parity I see between the struggles of those kids and the struggle of a physician trying to move through a 60-year medical history in a clunky interface in time to make a good decision for an anxious patient. I’m inspired by that struggle, which all of us face to a greater degree every day.
How does UCF empower you to do your research? UCF provides access to a brilliant community of students and collaborators. Industrial engineering is a friendly and collaborative faculty. I’ve met so many fascinating students in my classes and have been privileged to have some of them join my research group. I have graduated a few of these as scientists and engineers. I love the diversity at UCF: people from every imaginable walk of life are on this campus, and the perspectives they bring to this research make it possible. Moreover, I like the people I get to work with, and I wake up every day happy to see them and excited to move our work forward. I feel very lucky in this.
What major grants and honors have you earned to support your research? My readability research is primarily funded by industry. The consortium’s founding members Adobe and nonprofit Readability Matters provided the initial foundation for a community that now notably includes Google and Monotype. We also are beginning to work with these companies to attract state funding directly, including a 2023-24 $1 million appropriation from the State of Florida.
Why is this research important? Billions of readers have too much to read. The information age is only as miraculous as our individual abilities to access infinite information. The written word, one of the great engineering accomplishments of human history, was literally developed on reeds and animal hide. This research is founded in the idea that writing and reading, is due for an update. Rebuilding the written word to help humans of the information age is also an opportunity for languages that have not benefited so strongly from the digital revolution. Mandarin, Arabic, Hindi, Bengali and other scripts are underserved by modern Latin alphabet centered digital infrastructure but are receiving large investments as billions of these readers move online. Our readability research provides an opportunity to build equity in these languages, while working from evidence-based first principles of readability.
More Topics
Pegasus magazine.
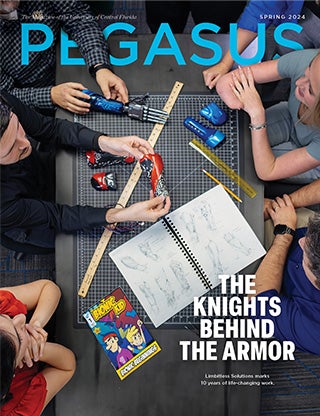
For a decade, UCF-based nonprofit Limbitless Solutions has transformed kids’ lives through bionic limbs.
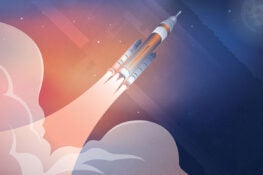
arXiv's Accessibility Forum starts next month!
Help | Advanced Search
Computer Science > Computation and Language
Title: assessing large language models for online extremism research: identification, explanation, and new knowledge.
Abstract: The United States has experienced a significant increase in violent extremism, prompting the need for automated tools to detect and limit the spread of extremist ideology online. This study evaluates the performance of Bidirectional Encoder Representations from Transformers (BERT) and Generative Pre-Trained Transformers (GPT) in detecting and classifying online domestic extremist posts. We collected social media posts containing "far-right" and "far-left" ideological keywords and manually labeled them as extremist or non-extremist. Extremist posts were further classified into one or more of five contributing elements of extremism based on a working definitional framework. The BERT model's performance was evaluated based on training data size and knowledge transfer between categories. We also compared the performance of GPT 3.5 and GPT 4 models using different prompts: naïve, layperson-definition, role-playing, and professional-definition. Results showed that the best performing GPT models outperformed the best performing BERT models, with more detailed prompts generally yielding better results. However, overly complex prompts may impair performance. Different versions of GPT have unique sensitives to what they consider extremist. GPT 3.5 performed better at classifying far-left extremist posts, while GPT 4 performed better at classifying far-right extremist posts. Large language models, represented by GPT models, hold significant potential for online extremism classification tasks, surpassing traditional BERT models in a zero-shot setting. Future research should explore human-computer interactions in optimizing GPT models for extremist detection and classification tasks to develop more efficient (e.g., quicker, less effort) and effective (e.g., fewer errors or mistakes) methods for identifying extremist content.
Subjects: | Computation and Language (cs.CL); Artificial Intelligence (cs.AI) |
Cite as: | [cs.CL] |
(or [cs.CL] for this version) | |
Focus to learn more arXiv-issued DOI via DataCite (pending registration) |
Submission history
Access paper:.
- Other Formats
References & Citations
- Google Scholar
- Semantic Scholar
BibTeX formatted citation

Bibliographic and Citation Tools
Code, data and media associated with this article, recommenders and search tools.
- Institution
arXivLabs: experimental projects with community collaborators
arXivLabs is a framework that allows collaborators to develop and share new arXiv features directly on our website.
Both individuals and organizations that work with arXivLabs have embraced and accepted our values of openness, community, excellence, and user data privacy. arXiv is committed to these values and only works with partners that adhere to them.
Have an idea for a project that will add value for arXiv's community? Learn more about arXivLabs .
- Skip to main content
- Skip to FDA Search
- Skip to in this section menu
- Skip to footer links

The .gov means it’s official. Federal government websites often end in .gov or .mil. Before sharing sensitive information, make sure you're on a federal government site.
The site is secure. The https:// ensures that you are connecting to the official website and that any information you provide is encrypted and transmitted securely.
U.S. Food and Drug Administration
- Search
- Menu
- News & Events
- FDA Newsroom
- Press Announcements
FDA Approves and Authorizes Updated mRNA COVID-19 Vaccines to Better Protect Against Currently Circulating Variants
FDA News Release
Today, the U.S. Food and Drug Administration approved and granted emergency use authorization (EUA) for updated mRNA COVID-19 vaccines (2024-2025 formula) to include a monovalent (single) component that corresponds to the Omicron variant KP.2 strain of SARS-CoV-2. The mRNA COVID-19 vaccines have been updated with this formula to more closely target currently circulating variants and provide better protection against serious consequences of COVID-19, including hospitalization and death. Today’s actions relate to updated mRNA COVID-19 vaccines manufactured by ModernaTX Inc. and Pfizer Inc.
In early June, the FDA advised manufacturers of licensed and authorized COVID-19 vaccines that the COVID-19 vaccines (2024-2025 formula) should be monovalent JN.1 vaccines. Based on the further evolution of SARS-CoV-2 and a rise in cases of COVID-19, the agency subsequently determined and advised manufacturers that the preferred JN.1-lineage for the COVID-19 vaccines (2024-2025 formula) is the KP.2 strain, if feasible.
“Vaccination continues to be the cornerstone of COVID-19 prevention,” said Peter Marks, M.D., Ph.D., director of the FDA’s Center for Biologics Evaluation and Research. “These updated vaccines meet the agency’s rigorous, scientific standards for safety, effectiveness, and manufacturing quality. Given waning immunity of the population from previous exposure to the virus and from prior vaccination, we strongly encourage those who are eligible to consider receiving an updated COVID-19 vaccine to provide better protection against currently circulating variants.”
The updated mRNA COVID-19 vaccines include Comirnaty and Spikevax, both of which are approved for individuals 12 years of age and older, and the Moderna COVID-19 Vaccine and Pfizer-BioNTech COVID-19 Vaccine, both of which are authorized for emergency use for individuals 6 months through 11 years of age.
What You Need to Know
- Unvaccinated individuals 6 months through 4 years of age are eligible to receive three doses of the updated, authorized Pfizer-BioNTech COVID-19 Vaccine or two doses of the updated, authorized Moderna COVID-19 Vaccine.
- Individuals 6 months through 4 years of age who have previously been vaccinated against COVID-19 are eligible to receive one or two doses of the updated, authorized Moderna or Pfizer-BioNTech COVID-19 vaccines (timing and number of doses to administer depends on the previous COVID-19 vaccine received).
- Individuals 5 years through 11 years of age regardless of previous vaccination are eligible to receive a single dose of the updated, authorized Moderna or Pfizer-BioNTech COVID-19 vaccines; if previously vaccinated, the dose is administered at least 2 months after the last dose of any COVID-19 vaccine.
- Individuals 12 years of age and older are eligible to receive a single dose of the updated, approved Comirnaty or the updated, approved Spikevax; if previously vaccinated, the dose is administered at least 2 months since the last dose of any COVID-19 vaccine.
- Additional doses are authorized for certain immunocompromised individuals ages 6 months through 11 years of age as described in the Moderna COVID-19 Vaccine and Pfizer-BioNTech COVID-19 Vaccine fact sheets.
Individuals who receive an updated mRNA COVID-19 vaccine may experience similar side effects as those reported by individuals who previously received mRNA COVID-19 vaccines and as described in the respective prescribing information or fact sheets. The updated vaccines are expected to provide protection against COVID-19 caused by the currently circulating variants. Barring the emergence of a markedly more infectious variant of SARS-CoV-2, the FDA anticipates that the composition of COVID-19 vaccines will need to be assessed annually, as occurs for seasonal influenza vaccines.
For today’s approvals and authorizations of the mRNA COVID-19 vaccines, the FDA assessed manufacturing and nonclinical data to support the change to include the 2024-2025 formula in the mRNA COVID-19 vaccines. The updated mRNA vaccines are manufactured using a similar process as previous formulas of these vaccines. The mRNA COVID-19 vaccines have been administered to hundreds of millions of people in the U.S., and the benefits of these vaccines continue to outweigh their risks.
On an ongoing basis, the FDA will review any additional COVID-19 vaccine applications submitted to the agency and take appropriate regulatory action.
The approval of Comirnaty (COVID-19 Vaccine, mRNA) (2024-2025 Formula) was granted to BioNTech Manufacturing GmbH. The EUA amendment for the Pfizer-BioNTech COVID-19 Vaccine (2024-2025 Formula) was issued to Pfizer Inc.
The approval of Spikevax (COVID-19 Vaccine, mRNA) (2024-2025 Formula) was granted to ModernaTX Inc. and the EUA amendment for the Moderna COVID-19 Vaccine (2024-2025 Formula) was issued to ModernaTX Inc.
Related Information
- Comirnaty (COVID-19 Vaccine, mRNA) (2024-2025 Formula)
- Spikevax (COVID-19 Vaccine, mRNA) (2024-2025 Formula)
- Moderna COVID-19 Vaccine (2024-2025 Formula)
- Pfizer-BioNTech COVID-19 Vaccine (2024-2025 Formula)
- FDA Resources for the Fall Respiratory Illness Season
- Updated COVID-19 Vaccines for Use in the United States Beginning in Fall 2024
- June 5, 2024, Meeting of the Vaccines and Related Biological Products Advisory Committee
The FDA, an agency within the U.S. Department of Health and Human Services, protects the public health by assuring the safety, effectiveness, and security of human and veterinary drugs, vaccines and other biological products for human use, and medical devices. The agency also is responsible for the safety and security of our nation’s food supply, cosmetics, dietary supplements, radiation-emitting electronic products, and for regulating tobacco products.
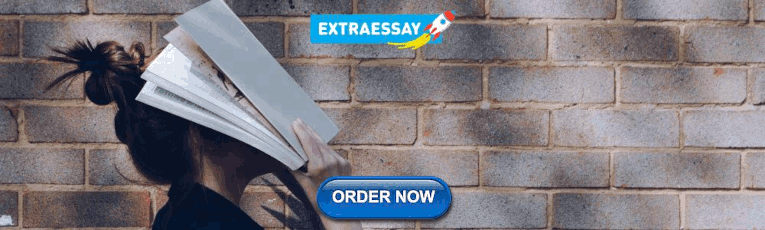
IMAGES
VIDEO
COMMENTS
This paper discusses the economic and philosophical inadequacies that have characterised the Project Tiger scheme in India. Launched in the 1970s to protect the habitats of the Royal Bengal Tiger, Project Tiger has over time evolved into a management system that has abstracted the tiger from its habitat by highlighting its charismatic functions.
This was an offer few forest dwellers could spurn, and many forest villages voluntary agreed to relocate outside the core. Project Tiger has since spent an average of 12.88 (SE 1.7) million USD per year for resettling people from core areas of tiger reserves to create inviolate space for tigers and their ecosystems (Figure S1). This monetary ...
Tapan Dutta. Project Tiger was conceived in the nascent seventies, out of concern for India's dwindling wilderness. Its contribution has been substantial. Moreover, the journey through these years has been one of significant learning, providing an insight into the involved linkages between nature conservation and sustainable community development.
The major purpose of the paper is to document the various research efforts and identify gaps that will need to be addressed in future interventions for the long-term monitoring and conservation of these predators, prey, and their habitats. ... the Namdapha TR and Kamlang TR falls under the umbrella of Project Tiger, which is a national tiger ...
Beyond borders, India's conservation commitment expands with the International Big Cat Alliance, launched to commemorate 'Project Tiger's' 50th anniversary. This initiative focuses on safeguarding iconic big cat species, spanning tigers, lions, leopards, snow leopards, pumas, jaguars, and cheetahs, engaging 97 countries with a ...
Paper statistics. If you need immediate assistance, call 877-SSRNHelp (877 777 6435) in the United States, or +1 212 448 2500 outside of the United States, 8:30AM to 6:00PM U.S. Eastern, Monday - Friday. The primary focus of the paper is to analyze the Indian Tiger Conservation program - Project Tiger. The circumstances that lead to and the ...
roj. ct Tiger: A Conservation Program for Saving the Indian TigerDR. CHITRA SINGH Department of Zool. la Thoburn College Lucknow-226007, Uttar Pradesh, IndiaAbstract:Project Tiger is a tiger conservation programme launched in 1973 by the. Government of India during Prime Minister Indira Gandhi's tenure. The project aims at ensuring a viable ...
Abstract. The primary focus of the paper is to analyze the Indian Tiger Conservation program - Project Tiger. The circumstances that lead to and the conditions under which the program was launched ...
Although Project Tiger was launched in 1973 to save India's wild tigers from the brink of extinction, the National Tiger Conservation Authority (NTCA) was established in 2005 to oversee and ...
The number of tigers in India has increased by 6.74 per cent from 2,967 in 2018 to 3,167 in 2022, according to the figures of the 5th cycle of India's Tiger Census, which was released by Prime Minister Narendra Modi at an event in Karnataka's Mysuru to mark 50 years of 'Project Tiger' on Sunday (April 9).. The PM also released the government's vision for tiger conservation during ...
Research Papers. Camera trap placement for evaluating species richness, abundance, and activity ... Research Papers; Tiger Reports; Contact Us. Prof. Qamar Qureshi, Wildlife Institute Of India (WII), Chandrabani, Dehradun - 248001, Uttarakhand. mstripes[dot]team[at]wii[dot]gov[dot]in
Project Tiger was meant to help save the tiger, and through protecting the apex predator, the larger ecosystem. But a 2020 stud y found that tigers have dominated research attention since the country's independence, and this has come at the cost of less charismatic species such as the desert fox, desert cat, striped hyena and the Indian wolf ...
The paper argues that tiger conservation has been a mix of sovereign, disciplinary and neoliberal environmentalities, all built on a certain 'truth' about tigers. The paper raises questions and concerns about the 'truth' discourse that underlies tiger conservation and also argues that tiger conservation has marginalized the ...
Project Tiger is a wildlife conservation movement initiated in India to protect the endangered tiger.The project was initiated in 1973 by the Ministry of Environment, Forest and Climate Change of the Government of India.As of March 2024, there are 55 protected areas that have been designated as tiger reserves under the project. As of 2023, there were 3,682 wild tigers in India, which is almost ...
It mentions The Project Tiger, launched by the Government of India in 1973 to ensure Bengal tigers' population and maintain their natural habitats, including setting up a tiger protecting force. The paper further examines the effectiveness of India's forest and wildlife laws.
By increasing forest cover, tiger conservation projects helped prevent the emission of at least 1 million metric tons of carbon dioxide between 2007 and 2020, the study reports. During this period ...
Anup Kumar Nayak, member secretary of the National Tiger Conservation Authority (NTCA), India's tiger conservation and research coordinating body, says that his agency has permitted several ...
The tiger is the key species and natural indicator of the health ... This work was supported by the Habitat Restoration Pilot Program II of Amur Tiger and Amur Leopard Project; and the Fundamental Research Funds for the Central Universities [2572016AA45]. ... H.D. is responsible for paper writing and data processing; Q.L. and J.Y. are ...
The Project Tiger is administered by The National Tiger Conservation Authority (NTCA). It is a statutory body of the Ministry with an overall supervisory and coordination part, performing capacities as gave in the Wildlife (Protection) Act, 1972. Project Tiger. The Project Tiger launched in 1973 is a 100% centrally sponsored scheme.
A tiger tissue sample was obtained from Satpura Tiger Reserve where the tiger died due to natural causes (was killed by another tiger in a territorial strife). This research project was conceived and radio collaring reported in this paper was done prior to the formation of an animal ethics committee at the Wildlife Institute of India.
One of the world's fiercest apex predators was locked in the battle of its life—and it was losing. At the turn of the 20th century, 100,000 tigers roamed the wilds of Asia, with India home to roughly 40,000 of the beasts. By 1972, that number had dropped to just 1,827. Determined not to let the tiger exist only in zoos, India's government launched an ambitious project in 1973 to establish ...
The number of tiger reserves in India has grown from 9 in 1973 to 50 in 2016 covering an area of 71027.10 SqKm (MoEF & CC, GoI, 2020). Tigers are not social animals; they need large undisturbed ...
Alexander Rybin, a long-term field technician for the Siberian Tiger Project, finished his Master's degree on Far Eastern leopards in June 2008 (with Dale Miquelle as one of his advisors), and will begin research for his Candidate of Science degree this fall. Alexander also works full-time as field crew leader for our leopard research project ...
The South China tiger (Panthera tigris amoyensis) is a tiger subspecies unique to China and one of the top ten endangered species in the world. It used to play an important role in the overall function of the ecosystem. This study rationally screened out key prey species of the South China tiger—the Chinese serow, Chinese goral, tufted deer, water deer, Chinese muntjac, red muntjac, sambar ...
This fact check originally appeared on PolitiFact. Project 2025 has a starring role in this week's Democratic National Convention. And it was front and center on Night 1. WATCH: Hauling large ...
My present research centers around human performance in reading: how can we best move information into your awareness, so you can do something with it. Are you a faculty member or student conducting research at UCF? We want to hear from you! Tell us about your research at bit.ly/ucf-research-60-form. Who inspires you to conduct your research?
We perform a critical examination of the scientific methodology behind contemporary large language model (LLM) research. For this we assess over 2,000 research works based on criteria typical of what is considered good research (e.g. presence of statistical tests and reproducibility) and cross-validate it with arguments that are at the centre of controversy (e.g., claims of emergent behaviour ...
View a PDF of the paper titled Assessing Large Language Models for Online Extremism Research: Identification, Explanation, and New Knowledge, by Beidi Dong and 3 other authors View PDF Abstract: The United States has experienced a significant increase in violent extremism, prompting the need for automated tools to detect and limit the spread of ...
The SEI Digital Library provides access to more than 6,000 documents from three decades of research into best practices in software engineering. These documents include technical reports, presentations, webcasts, podcasts and other materials searchable by user-supplied keywords and organized by topic, publication type, publication year, and author.
FDA approved and authorized for emergency use updated mRNA COVID-19 vaccines (2024-2025 formula) to more closely target currently circulating variants to prevent COVID-19 and to provide better ...