- Investors External
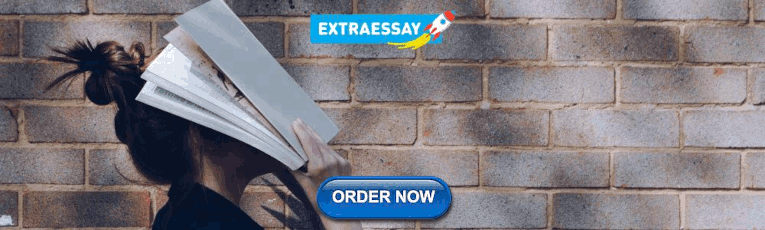
Lab Experiment 3: Gamma-Ray Absorption in Matter (Basic)
- Share to Facebook
- Share to Twitter
- Share to Linkedin
- To demonstrate attenuation of gamma rays in matter.
Equipment Required:
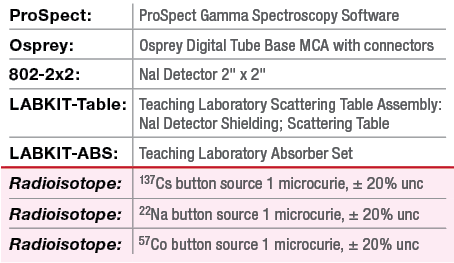
Theoretical Overview:

Gamma-ray interactions with matter
There are three dominant gamma-ray interactions with matter:
Photoelectric effect
- Compton effect
Pair production
The photoelectric effect is a common interaction between a low-energy photon and a material. In this process the photon interacts with an electron in the material losing all of its energy. The electron is ejected with an energy equal to the initial photon energy minus the binding energy of the electron. This is a useful process for spectroscopy since an output pulse in a detector is produced that is proportional to the gamma-ray energy, as all of the energy of the gamma ray is transferred to the detector. This produces a characteristic full-energy peak in the spectrum that can be used for the purpose of identifying the radioactive material.
The probability of the photoelectric effect is strongly dependent on the Z of the atoms in the matter and the photon energy; it is the dominant process at low photon energy. The probability has discontinuities at the binding energies of the electrons in the constituent atoms in the matter because the probability of transferring the energy to an electron with higher binding energy than the incoming photon energy is zero. The probability of the photoelectric effect therefore rapidly decreases when transitioning from a photon energy just above the binding energy of the electrons to an energy just below it.
Compton scattering
In the Compton effect, the gamma ray scatters from an electron, transferring an amount of energy that depends upon the angle of scatter.
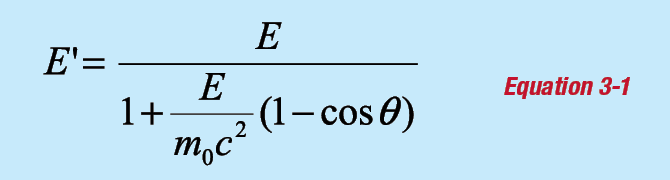
where: E' is the scattered energy of the gamma ray. E is the incident gamma-ray energy. θ is the angle of scatter.
The term m 0 c 2 is the rest mass of the electron, equal to 511 keV. The energy given to the electron is:

The maximum energy given to an electron in Compton scattering occurs for a scattering angle of 180˚, and the energy distribution is continuous up to that point (since all scattering angles up to 180˚ are possible).
If the photon is on a trajectory towards the detector it can Compton scatter at a small angle and still result in hitting the detector. However, since all Compton scattering transfers some energy to the electron, the scattered photon will not deposit its full initial energy in the detector and it will not contribute to the full-energy peak. In the vast majority of measurements performed with spectroscopic detectors the quantity that is measured is the number of counts in the full-energy peak so a Compton-scattered photon is considered to be an unwanted photon even if it ends up depositing energy in the detector.
Pair production can occur when the gamma-ray energy is greater than 1.022 MeV and is a significant process at energies above 2.5 MeV. The process produces a positron and electron pair that slows down through scattering interactions in the matter. When the positron comes to rest it annihilates with an electron producing a pair of 511 keV gamma rays that are emitted back-to-back.
The probability of pair production is 0 up to the energy threshold of twice the electron mass (1.022 MeV/c 2 ) and it increases with energy up to 100 MeV where it becomes constant.
The total photon interaction probability in matter
Figure 3-1 shows the probability of the three dominant interaction processes in matter as a function of photon energy. The figure displays the discontinuity at the binding energy of the electrons in the atom, the decrease of the probability of photoelectric effect when the energy increases, the dominance of Compton scattering at medium energies and the dominance of pair production at higher photon energies.
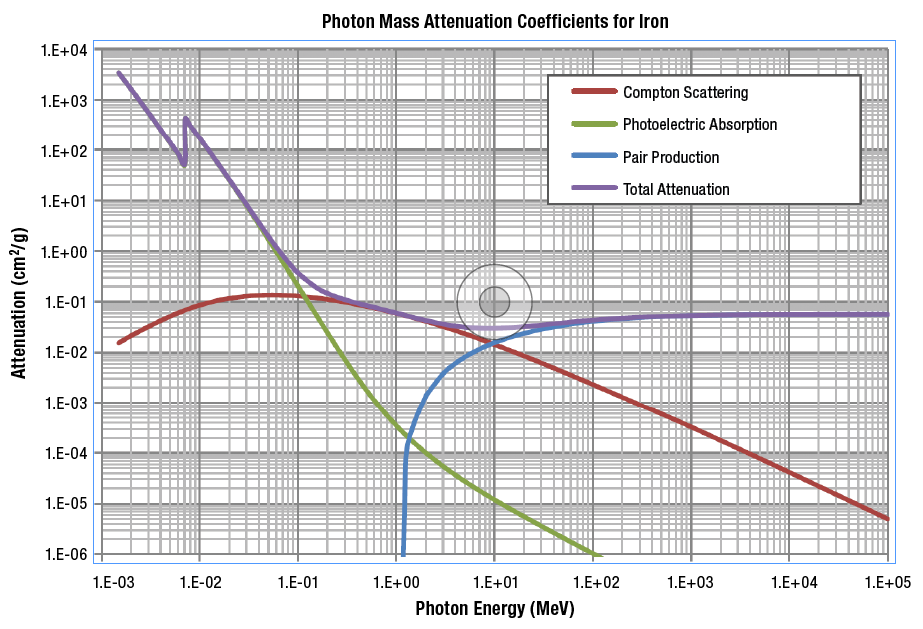
Figure 3-1: The probability of photon interaction as a function of energy
Attenuation in matter
The rate of change per unit length (dI/dx) of photons of intensity I traversing matter is given by:

where µ is the linear attenuation coefficient and this is dependent upon the proton (Z) number of the attenuating material, the material density and the photon energy.
By solving this differential equation, the intensity as a function of the length traversed I(x) can be obtained:

where I 0 is the initial intensity at x=0.
Half-length
The half-length x 1/2 is defined as the length at which the photon intensity is reduced to one-half. It can be expressed as:

And solving for x 1/2 gives:

This means that as the attenuation of a material increases, the half-length gets shorter.
Experiment 3 Guide:
1. Ensure that the Osprey (with the NaI(Tl) detector connected) is connected to the measurement PC either directly or via your local network.
2. Place the 137 Cs source in front of the detector.
3. Open the ProSpect Gamma Spectroscopy Software and connect to the Osprey.
4. Configure your detector settings as recommended in Experiment 1.
5. Use the software to apply the recommended detector bias to the NaI(Tl) detector.
6. Set the amplifier gain such that the full-energy peak is close to one third of the spectrum.
7. Acquire a spectrum (use a count time such that there is at least 10 000 counts in the full-energy peak).
8. Make a record of the number of counts in the full-energy peak and the count time.
9. Place one piece of the aluminum attenuator between the source and the detector.
10. Acquire a spectrum (use a count time such that there is at least 10 000 counts in the full-energy peak or acquire for 5 minutes, whichever is shortest).
11. Make a record of the number of counts in the full-energy peak, the count time and the thickness of the absorber.
12. Add another piece of aluminum attenuation and repeat steps 10 and 11.
13. Repeat step 12 until there are 5 absorbers between the source and the detector.
14. Repeat step 9-13 for lead and polyethylene.
15. Repeat step 9-13 for one of the attenuator materials for a 22 Na source and a 57 Co source.
16. Using Microsoft Excel or another graphing application, plot the count rate (number of counts in the full-energy peak divided by the count time) for 137 Cs for the three absorbers. Do the graphs have the expected shapes? Which material attenuates photons most?
17. Plot the count rate as a function of attenuator thickness for the three sources. Which source has the highest penetration through matter?
18. Using one of the plots, read out the half-length thickness for that material and energy and calculate the linear attenuation coefficient.
Related Articles
Genie™ 4.0 and apex ® products operating system and database qualifications.
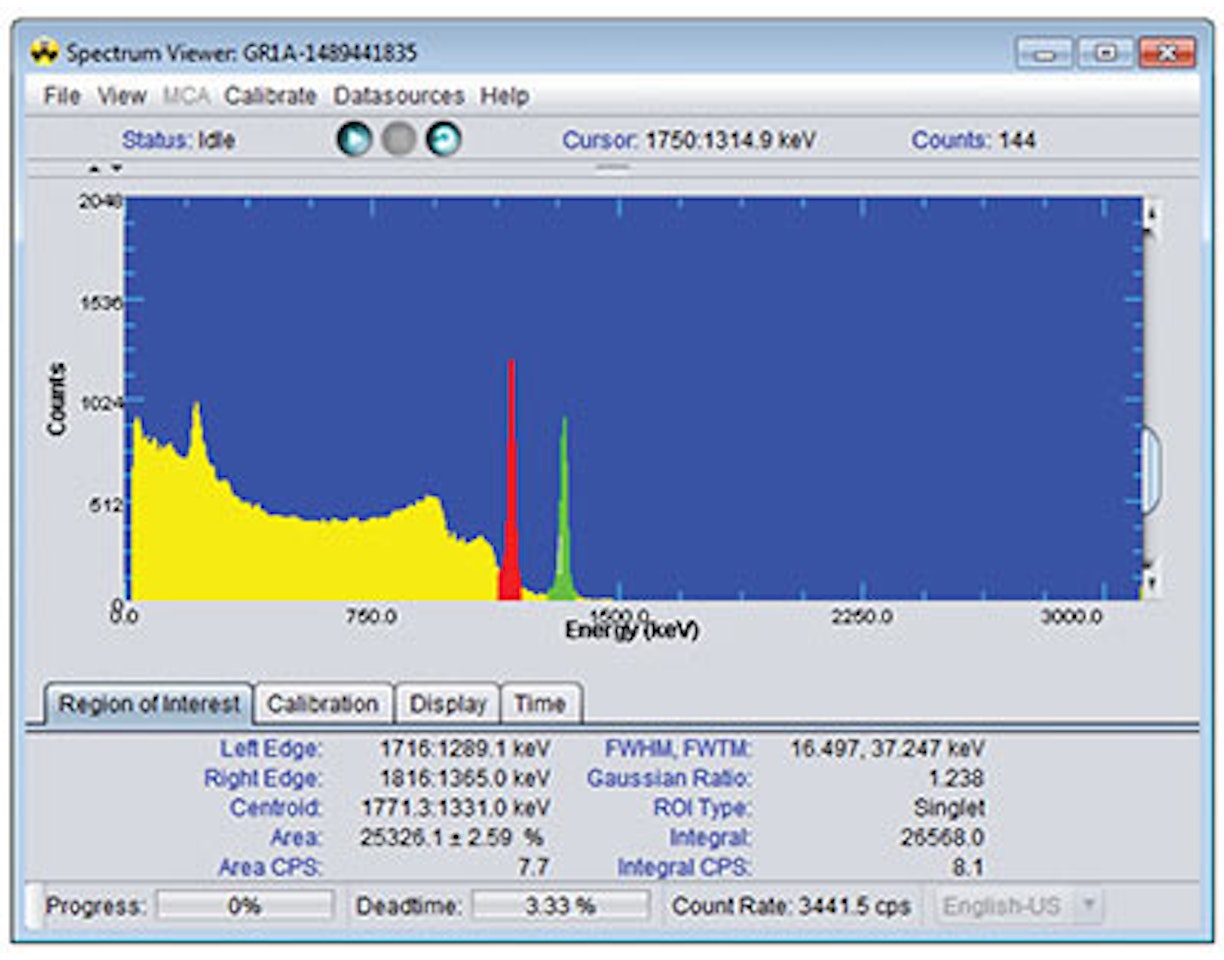
Software Options for GR1™, Sigma™, and TN-15™ Family Devices
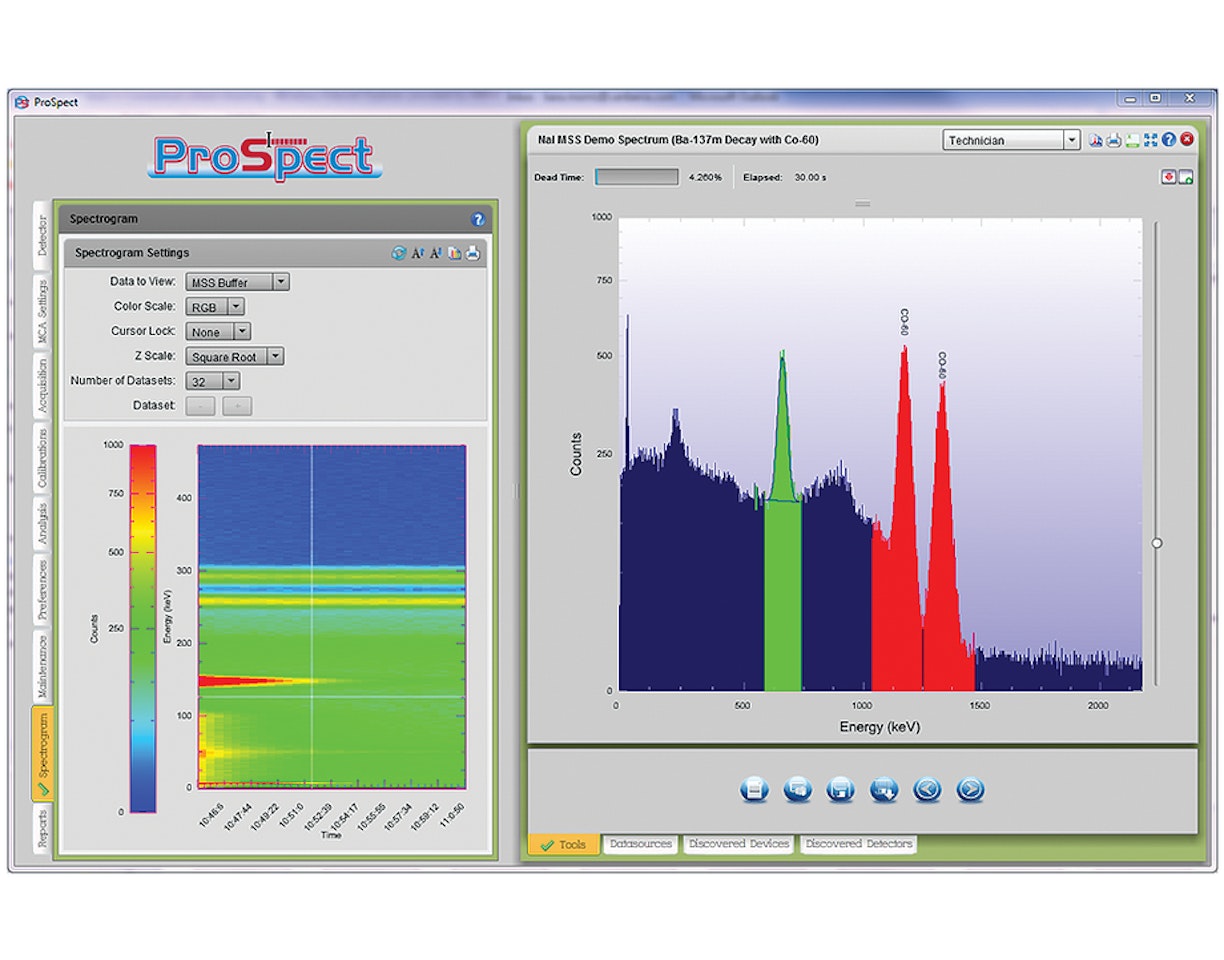
ProSpect TLIST Data Scanner
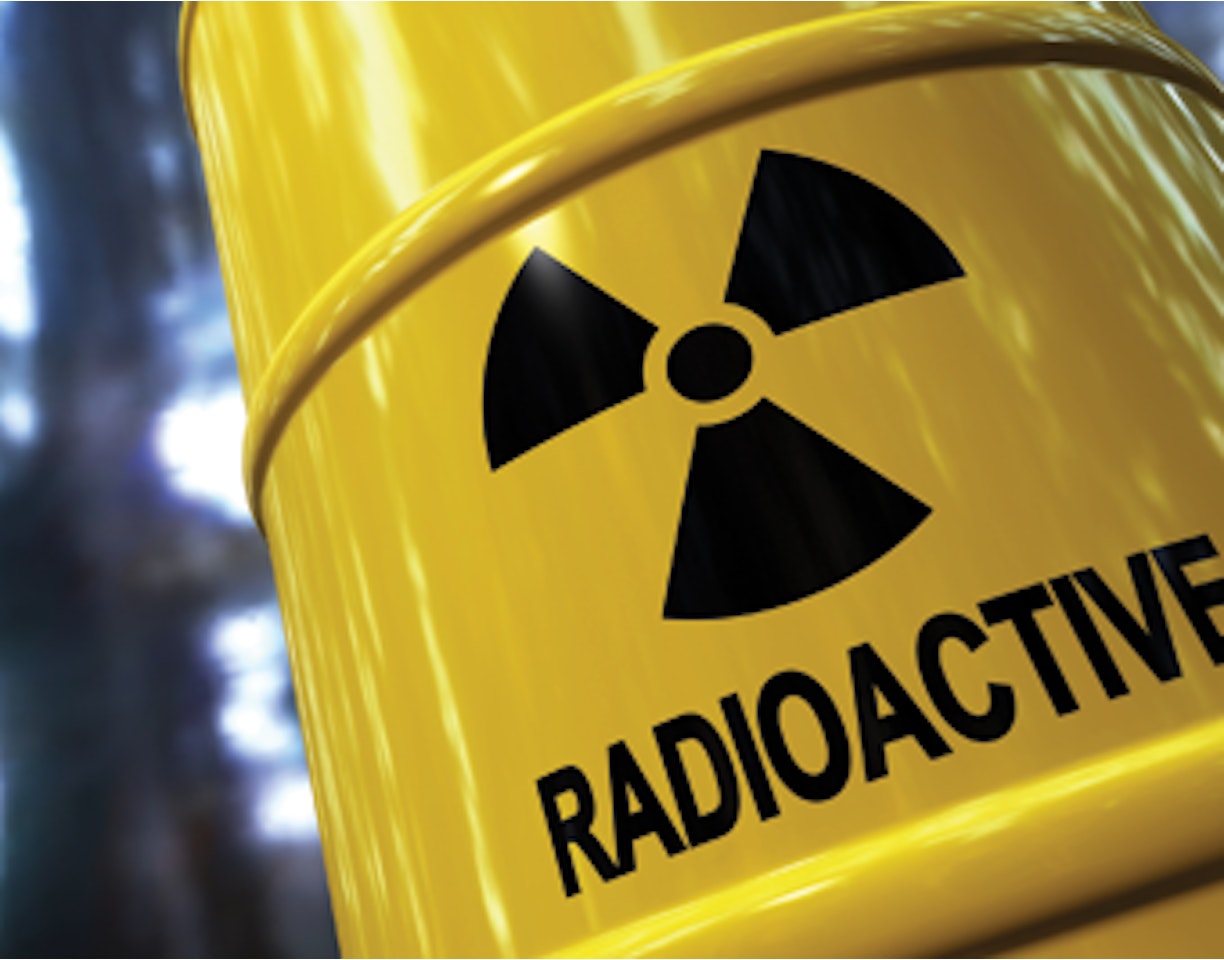
What is Radiation?
Before exploring the various ways that radiation is used in and affects the world around you, it’s important to have a general understanding of what radiation is and how it works on a fundamental level.
Looking for Services or Support? We're here to help.
Experiment 4. GAMMA-RAY ABSORPTION
Our systems are now restored following recent technical disruption, and we’re working hard to catch up on publishing. We apologise for the inconvenience caused. Find out more: https://www.cambridge.org/universitypress/about-us/news-and-blogs/cambridge-university-press-publishing-update-following-technical-disruption
We use cookies to distinguish you from other users and to provide you with a better experience on our websites. Close this message to accept cookies or find out how to manage your cookie settings .
Login Alert
- > Atomic Structure and Lifetimes
- > Absorption of radiation
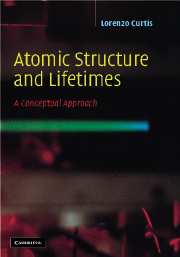
Book contents
- Frontmatter
- List of physical constants and useful interrelations
- 1 Introduction
- 2 Semiclassical conceptual models
- 3 Semiempirical parametrization of energy-level data
- 4 The vector model of angular momentum
- 5 The intermediate coupling model
- 6 Electric dipole radiation
- 7 Line strengths in two-valence-electron systems
- 8 Magnetic dipole transitions
- 9 Absorption of radiation
- 10 Time-resolved measurements
- 11 Hyperfine structure
- 12 Electrostatic polarizabilities and long-range interactions
- 13 Coherence and anisotropic excitation
9 - Absorption of radiation
Published online by Cambridge University Press: 06 July 2010
Backward, turn backward, O time in your flight. Make me a child again, just for tonight.
Since the time of Kirchhoff it has been known that, when light is passed through an atomic gas, those wavelengths are observed that would be emitted if the gas were incandescent. If the gas is sufficiently cold, then the wavelengths observed are limited to ground-state transitions. As the temperature of the sample is elevated, wavelengths corresponding to transitions between excited states become absorbing, and balances between emission and absorption occur.
The study of the central wavelengths of emission lines or absorption notches is known as first-order spectroscopy, and it provides information on the energy-level structure of the atom. The study of the shape of these lines in frequency space is known as second-order spectroscopy, and provides information on the lifetime of the level and the collision rates and temperature of the gas. Thus, whereas first-order spectroscopy shows that emission and absorption measurements yield the same central wavelengths, second-order spectroscopy shows that the natural linewidth for emission and absorption are both specified by the level lifetime, and that the intensity of emission and absorption features both involve the linestrength factor (through the emission transition probability rate and the absorption oscillator strength).
The connection between the lifetime and the linewidth can be made plausible by a simple semiclassical model. When an electron is excited to a specific orbit in an atom, its binding energy is established through the exchange of virtual photons with the effective central core. If the perturbations that eventually cause the electron to make a transition from that orbit are weak, the meanlife will be long.
Access options
Save book to kindle.
To save this book to your Kindle, first ensure [email protected] is added to your Approved Personal Document E-mail List under your Personal Document Settings on the Manage Your Content and Devices page of your Amazon account. Then enter the ‘name’ part of your Kindle email address below. Find out more about saving to your Kindle .
Note you can select to save to either the @free.kindle.com or @kindle.com variations. ‘@free.kindle.com’ emails are free but can only be saved to your device when it is connected to wi-fi. ‘@kindle.com’ emails can be delivered even when you are not connected to wi-fi, but note that service fees apply.
Find out more about the Kindle Personal Document Service .
- Absorption of radiation
- Lorenzo J. Curtis , University of Toledo, Ohio
- Book: Atomic Structure and Lifetimes
- Online publication: 06 July 2010
- Chapter DOI: https://doi.org/10.1017/CBO9780511755552.010
Save book to Dropbox
To save content items to your account, please confirm that you agree to abide by our usage policies. If this is the first time you use this feature, you will be asked to authorise Cambridge Core to connect with your account. Find out more about saving content to Dropbox .
Save book to Google Drive
To save content items to your account, please confirm that you agree to abide by our usage policies. If this is the first time you use this feature, you will be asked to authorise Cambridge Core to connect with your account. Find out more about saving content to Google Drive .
Rutherford's Experiment to Understand β-Rays
Nikolas martelaro february 20, 2017, submitted as coursework for ph241 , stanford university, winter 2017, introduction.
Experimental Setup to determine existence of β-rays. (Source: N. Martelaro) |
Scientific experiments are the foundation for building our understanding of the physical world. While experiments may seem complex to the non-scientist, in reality, many influential scientific discoveries have been made with beautifully simple experiments. One such example is Earnest Rutherford's experiment on the nature of uranium radiation and its ability to pass through various materials. [1] Using the fact that the radiation from Uranium ionizes a gas, thus creating charges particles, Rutherford was able to measure the current produced by a sample of uranium placed between two charged metal plates. By placing metal foils on top of the uranium, Rutherford showed that part of the ionizing radiation was stopped while another part appeared to pass through the foils. This helped to confirm the work of Becquerel, showing that there were two components of the ionizing radiation. [2] These two rays were given the names α-rays and β-rays. While Becquerel's works suggested the existence of the two types of rays, there was still little understanding of their nature. Specifically, through how much and what types of material did these rays pass through? Rutherford aimed to explore this using his simple and beautiful experiment and was able to show the differences between α and β radiation absorption. This experiment would later lead to him advising Geiger and Mardsen's famous gold foil experiment, whereupon α particles moving through gold foil were shown to sometimes deflect, suggesting the positive nuclear core model of the atom that we know today. [3] This report gives an overview of the design and results of Rutherford's experiment, which is described in detail in his 1899 paper. [1] It should be noted that Rutherford details a number of experiments in his paper, only the experiment that confirmed the existence of α and β rays will be discussed here.
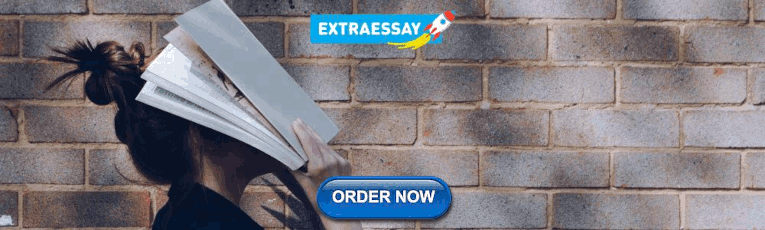
Known Theory of Uranium Radiation
At the time of Rutherford's experiment, uranium was know to emit an ionizing radiation similar to x-rays. When subjected to a gas, this radiation would create positively and negatively charged particles. This allows the gas to be a temporary conductor of electricity and would allow an electric potential and current to be measured. From Becquerel's work, it was known that the radiation would penetrate solid material, but it was not known through how much material. It was also known that the rays emitted from uranium had varying powers.
From this theory, Rutherford hypothesized that the rays emitting from uranium would be complex, composed of different types of rays. He proposed that testing how well the rays penetrated metal foils may help to show what the characteristics of the rays were.
Experiment Setup
Rutherford's experimental setup was quite simple. A diagram of it is shown in Fig. 1. A sample of uranium is placed between two plates A and B. Plate A is charged by a battery to 50 V, while plate B is connected to the sensing element of an electrometer. This creates an electrical potential between the plates. Due to the ionizing radiation coming from the uranium, the gas in between the plates will become electrically charged. Positive ions will move away from plate A while negative ion will move toward it. This will induce a small current between the plates. As this current flows in the gas, it will create a charge on the sensing element of the electrometer.
The electrometer works by having four separated quadrants. The quadrants are hollow inside, much like a bicycle tire without a tube. The diagonally opposing quadrants are connected together electrically. One set of quadrants is connected to earth ground while the other acts as the sensing quadrant and is connected to Plate B. A metal vane (or needle) is placed inside this hollow area of the quadrants. The vane hangs from a thread, allowing it to spin inside of the quadrants. The vane is then charged. When a charge is induced on Plate B and the sensing quadrant of the electrometer, it begins to spin the vane due to the opposing electrical forces, similar to how magnets with the north poles facing each other will repel each other. The degree that the vane turns is associated with the voltage, while the rate that the vane turns is associated with the current.
To explore the nature of the radiation, Rutherford covered the uranium with a thin sheet of metal foil. He then measured the "rate of leak" given by the electrometer vane when in constant motion (indicating a specific amount of current, and thus an amount of charge induced by the ionizing radiation). By placing successive sheets of foil, Rutherford was able to see how much the rate of leak diminished, indicating how much of the radiation was blocked.
COMMENTS
Learn how different colors affect the amount of radiant energy absorbed and re-emitted by objects, and how to calculate the rate of energy flow using the Stefan-Boltzmann equation. This physics science fair project uses an infrared thermometer to measure the temperature of colored paper and compare it with black and white squares.
Demonstration: Absorption of radiation by living matter. To simulate the absorption of radiation by living matter use slices of different vegetables as absorbers, or a slice of bacon to represent human flesh. Episode 511-5: Absorption in biological materials (Word, 53 KB) Student experiments: Optional. The first requires a sealed radium-226 source.
HB 01-2102-02 Absorption Of Radiation (1) Lab 4 1 Absorption Of Radiation (1) Lab 4 1 Purpose In this experiment the absorption of beta ( =negative electrons) and gamma (=high energy photons) radiation by various materials is studied. Measurements are done with a Geiger-Muller tube and scaler. 2 Geiger-Muller Counter The Geiger-Muller tube (the ...
PHYS 1493/1494/2699: Exp. 10 - Absorption of β and γ Rays 2 Introduction Background: Radioactivity and nuclear decay (α, β, γ) The experiment: Detecting β rays: the Geiger-Müller counter Finding the plateau region (optimal voltage) Counting statistics: Poisson statistics Measurements: Estimating background Determining β-rays range and energy
Experiment 3 Guide: 1. Ensure that the Osprey (with the NaI(Tl) detector connected) is connected to the measurement PC either directly or via your local network. 2. Place the 137 Cs source in front of the detector. 3. Open the ProSpect Gamma Spectroscopy Software and connect to the Osprey. 4. Configure your detector settings as recommended in ...
How William Wilson challenged the prevailing view that beta rays (electrons) were monoenergetic and followed an exponential absorption law in matter. Learn about his experiments, his impact on the field, and his disappearance from the physics literature.
Experiment 4. GAMMA-RAY ABSORPTION In this experiment you will measure the transmission of gamma rays through different absorbers. Theoretically, there should be an exponential decrease of the transmitted counts with thickness of absorber, determined by a mass absorption coefficient. ... Background radiation may come from cosmic rays and ...
Learn how matter absorbs photons and transforms them into internal energy. Find out how absorption affects different fields and applications, such as radio, meteorology, medicine, chemistry, optics, and biology.
EXPERIMENT 2 -10 ABSORPTION OF BETA AND GAMMA RAYS Objective: To study the behavior of gamma and beta rays passing through matter; to measure the range of beta-particles from a given source and hence to determine the endpoint energy of decay; to determine the absorption coefficient in lead of the gamma radiation from a given source.
Download the full set of Q&A flashcards for this course at: https://gcsephysicsninja.com/lessons/thermal-physics/This tutorial explains how the amount of inf...
The study of the central wavelengths of emission lines or absorption notches is known as first-order spectroscopy, and it provides information on the energy-level structure of the atom. The study of the shape of these lines in frequency space is known as second-order spectroscopy, and provides information on the lifetime of the level and the ...
Thus, the absorption by matter of gamma radiation greater than 1.02 MeV may result in pair production. The probability of pair production increases in proportion to the magnitude of gamma-ray photon energy above 1.02 MeV, and pair production is the predominant mechanism of the absorption of photons of energies of 5 MeV and above (see Figs. 13. ...
Learn how Rutherford used metal foils to show the differences between α and β radiation from uranium. See how aluminum foil blocked most of the β-rays, while uranium radiation could pass through it.
Learn about the techniques and applications of measuring the absorption of electromagnetic radiation by a sample as a function of frequency or wavelength. Find out how absorption spectra are related to emission, transmission, scattering and reflection spectra, and how they depend on the atomic and molecular structure of the sample.
Learn how to measure the transmission of beta particles through materials using a 90 S r source and a GM tube. Find the absorption coefficient and the half-thickness of the material from the exponential fit of the data.
This simple experiment provides a quick demonstration of the effect of colour on the absorption off thermal radiation.Two identical beaks are painted differe...
A high school science GCSE iGcse physics revision video all about absorption of radiation emitted by radioactive nuclei. Alpha, Beta and Gamma radiation and ...
358 BureauojStandardsJournalofResearch [Vol.8 continuedtouseit.Figure1istakenfrompaperNo.371,andit showstheemissionintensitydistributionandtheabsorptioncurves ...
Learn how to calculate the spectral absorption coefficient and the radiative lifetime of a gas using Einstein coefficients of radiation. See how the eqn. of radiative transfer applies to different types of emission and absorption experiments.
Students use a Geiger counter to measure the radiation from a radioactive sample of Sr-90. They use the radiation absorption equation to predict the counts after one aluminum shield is used and find the counts are much higher than the prediction. After hypothesizing reasons why, they learn it is because the daughter product of Sr-90, Y-90, is radioactive and has higher energy beta particles ...
A set of flashcards for a lab experiment on how different materials and angles of light affect the absorption of heat from the sun. The answer to the query is that the more direct light a material receives, the more heat it absorbs.