The double-slit experiment: Is light a wave or a particle?
The double-slit experiment is universally weird.
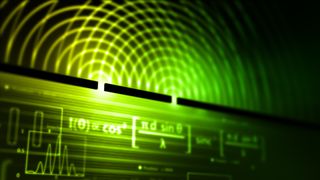
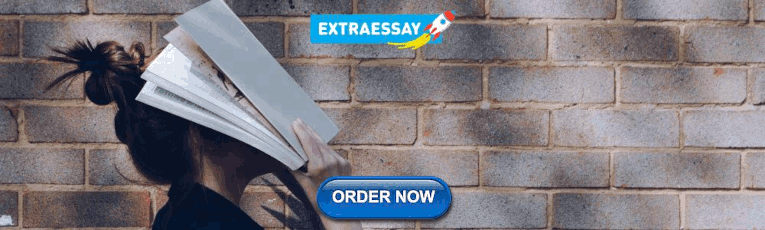
How does the double-slit experiment work?
Interference patterns from waves, particle patterns, double-slit experiment: quantum mechanics, history of the double-slit experiment, additional resources.
The double-slit experiment is one of the most famous experiments in physics and definitely one of the weirdest. It demonstrates that matter and energy (such as light) can exhibit both wave and particle characteristics — known as the particle-wave duality of matter — depending on the scenario, according to the scientific communication site Interesting Engineering .
According to the University of Sussex , American physicist Richard Feynman referred to this paradox as the central mystery of quantum mechanics.
We know the quantum world is strange, but the two-slit experiment takes things to a whole new level. The experiment has perplexed scientists for over 200 years, ever since the first version was first performed by British scientist Thomas Young in 1801.
Related: 10 mind-boggling things you should know about quantum physics
Christian Huygens was the first to describe light as traveling in waves whilst Isaac Newton thought light was composed of tiny particles according to Las Cumbres Observatory . But who is right? British polymath Thomas Young designed the double-slit experiment to put these theories to the test.
To appreciate the truly bizarre nature of the double-split experiment we first need to understand how waves and particles act when passing through two slits.
When Young first carried out the double-split experiment in 1801 he found that light behaved like a wave.
Firstly, if we were to shine a light on a wall with two parallel slits — and for the sake of simplicity, let's say this light has only one wavelength.
As the light passes through the slits, each, in turn, becomes almost like a new source of light. On the far side of the divider, the light from each slit diffracts and overlaps with the light from the other slit, interfering with each other.
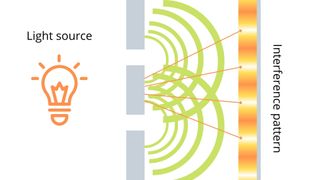
According to Stony Brook University , any wave can create an interference pattern, whether it be a sound wave, light wave or waves across a body of water. When a wave crest hits a wave trough they cancel each other out — known as destructive interference — and appear as a dark band. When a crest hits a crest they amplify each other — known as constructive interference — and appear as a bright band. The combination of dark and bright bands is known as an interference pattern and can be seen on the sensor screen opposite the slits.
This interference pattern was the evidence Young needed to determine that light was a wave and not a particle as Newton had suggested.
But that is not the whole story. Light is a little more complicated than that, and to see how strange it really is we also need to understand what pattern a particle would make on a sensor field.
If you were to carry out the same experiment and fire grains of sand or other particles through the slits, you would end up with a different pattern on the sensor screen. Each particle would go through a slit end up in a line in roughly the same place (with a little bit of spread depending on the angle the particle passed through the slit).
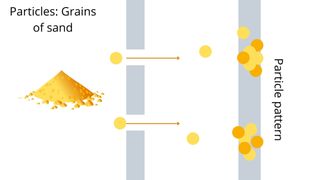
Clearly, waves and particles produce a very different pattern, so it should be easy to distinguish between the two right? Well, this is where the double-slit experiment gets a little strange when we try and carry out the same experiment but with tiny particles of light called photons. Enter the realm of quantum mechanics.
The smallest constituent of light is subatomic particles called photons. By using photons instead of grains of sand we can carry out the double-slit experiment on an atomic scale.
If you block off one of the slits, so it is just a single-slit experiment, and fire photons through to the sensor screen, the photons will appear as pinprick points on the sensor screen, mimicking the particle patterns produced by sand in the previous example. From this evidence, we could suggest that photons are particles.
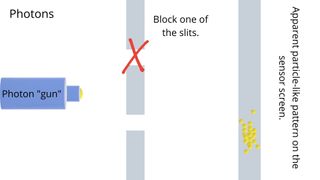
Now, this is where things start to get weird.
If you unblock the slit and fire photons through both slits, you start to see something very similar to the interference pattern produced by waves in the light example. The photons appear to have gone through the pair of slits acting like waves.
But what if you launch photons one by one, leaving enough time between them that they don't have a chance of interfering with each other, will they behave like particles or waves?
At first, the photons appear on the sensor screen in a random scattered manner, but as you fire more and more of them, an interference pattern begins to emerge. Each photon by itself appears to be contributing to the overall wave-like behavior that manifests as an interference pattern on the screen — even though they were launched one at a time so that no interference between them was possible.
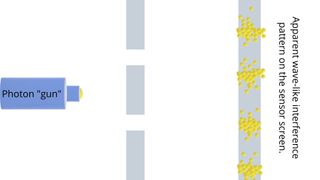
It's almost as though each photon is "aware" that there are two slits available. How? Does it split into two and then rejoin after the slit and then hit the sensor? To investigate this, scientists set up a detector that can tell which slit the photon passes through.
Again, we fire photons one at a time at the slits, as we did in the previous example. The detector finds that about 50% of the photons have passed through the top slit and about 50% through the bottom, and confirms that each photon goes through one slit or the other. Nothing too unusual there.
But when we look at the sensor screen on this experiment, a different pattern emerges.
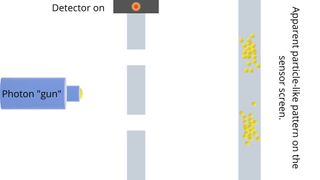
This pattern matches the one we saw when we fired particles through the slits. It appears that monitoring the photons triggers them to switch from the interference pattern produced by waves to that produced by particles.
If the detection of photons through the slits is apparently affecting the pattern on the sensor screen, what happens if we leave the detector in place but switch it off? (Shh, don't tell the photons we're no longer spying on them!)
This is where things get really, really weird.
Same slits, same photons, same detector, just turned off. Will we see the same particle-like pattern?
No. The particles again make a wave-like interference pattern on the sensor screen.
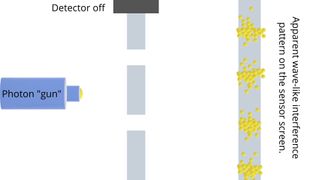
The atoms appear to act like waves when you're not watching them, but as particles when you are. How? Well, if you can answer that, a Nobel Prize is waiting for you.
In the 1930s, scientists proposed that human consciousness might affect quantum mechanics. Mathematician John Von Neumann first postulated this in 1932 in his book " The Mathematical Foundations of Quantum Mechanics ." In the 1960s, theoretical physicist, Eugene Wigner conceived a thought experiment called Wigner's friend — a paradox in quantum physics that describes the states of two people, one conducting the experiment and the observer of the first person, according to science magazine Popular Mechanics . The idea that the consciousness of a person carrying out the experiment can affect the result is knowns as the Von Neumann–Wigner interpretation.
Though a spiritual explanation for quantum mechanic behavior is still believed by a few individuals, including author and alternative medicine advocate Deepak Chopra , a majority of the science community has long disregarded it.
As for a more plausible theory, scientists are stumped.
Furthermore —and perhaps even more astonishingly — if you set up the double-slit experiment to detect which slit the photon went through after the photon has already hit the sensor screen, you still end up with a particle-type pattern on the sensor screen, even though the photon hadn't yet been detected when it hit the screen. This result suggests that detecting a photon in the future affects the pattern produced by the photon on the sensor screen in the past. This experiment is known as the quantum eraser experiment and is explained in more detail in this informative video from Fermilab .
We still don't fully understand how exactly the particle-wave duality of matter works, which is why it is regarded as one of the greatest mysteries of quantum mechanics.
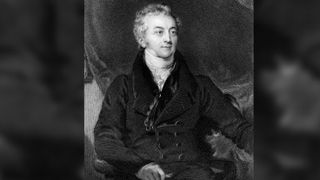
The first version of the double-slit experiment was carried out in 1801 by British polymath Thomas Young, according to the American Physical Society (APS). His experiment demonstrated the interference of light waves and provided evidence that light was a wave, not a particle.
Young also used data from his experiments to calculate the wavelengths of different colors of light and came very close to modern values.
Despite his convincing experiment that light was a wave, those who did not want to accept that Isaac Newton could have been wrong about something criticized Young. (Newton had proposed the corpuscular theory, which posited that light was composed of a stream of tiny particles he called corpuscles.)
According to APS, Young wrote in response to one of the critics, "Much as I venerate the name of Newton, I am not therefore obliged to believe that he was infallible."
Since the development of quantum mechanics, physicists now acknowledge light to be both a particle and a wave.
Explore the double-slit experiment in more detail with this article from the University of Cambridge, which includes images of electron patterns in a double-slit experiment. Discover the true nature of light with Canon Science Lab . Read about fragments of energy that are not waves or particles — but could be the fundamental building blocks of the universe — in this article from The Conversation . Dive deeper into the two-slit experiment in this article published in the journal Nature .
Bibliography
Grangier, Philippe, Gerard Roger, and Alain Aspect. " Experimental evidence for a photon anticorrelation effect on a beam splitter: a new light on single-photon interferences. " EPL (Europhysics Letters) 1.4 (1986): 173.
Thorn, J. J., et al. "Observing the quantum behavior of light in an undergraduate laboratory. " American Journal of Physics 72.9 (2004): 1210-1219.
Ghose, Partha. " The central mystery of quantum mechanics. " arXiv preprint arXiv:0906.0898 (2009).
Aharonov, Yakir, et al. " Finally making sense of the double-slit experiment. " Proceedings of the National Academy of Sciences 114.25 (2017): 6480-6485.
Peng, Hui. " Observations of Cross-Double-Slit Experiments. " International Journal of Physics 8.2 (2020): 39-41.
Join our Space Forums to keep talking space on the latest missions, night sky and more! And if you have a news tip, correction or comment, let us know at: [email protected].
Get the Space.com Newsletter
Breaking space news, the latest updates on rocket launches, skywatching events and more!
Daisy Dobrijevic joined Space.com in February 2022 having previously worked for our sister publication All About Space magazine as a staff writer. Before joining us, Daisy completed an editorial internship with the BBC Sky at Night Magazine and worked at the National Space Centre in Leicester, U.K., where she enjoyed communicating space science to the public. In 2021, Daisy completed a PhD in plant physiology and also holds a Master's in Environmental Science, she is currently based in Nottingham, U.K. Daisy is passionate about all things space, with a penchant for solar activity and space weather. She has a strong interest in astrotourism and loves nothing more than a good northern lights chase!
UAE on track to launch bold 7-asteroid mission in 2028
Space pictures! See our space image of the day
Belief in alien visits to Earth is spiraling out of control – here's why that's so dangerous
Most Popular
- 2 New 'StarRupture' gameplay trailer is a killer bug hunt on a crazy planet (video)
- 3 Should we regulate the moon? Scientists call for international plan to share lunar water and resources
- 4 UAE on track to launch bold 7-asteroid mission in 2028
- 5 Hubble telescope spies a sparkling 'cosmic fossil' 3 million light-years away (image)
December 12, 2013
Double-Slit Science: How Light Can Be Both a Particle and a Wave
Learn how light can be two things at once with this illuminating experiment
By Education.com & Mack Levine
Key Concepts Light Wave Particle Lasers
Introduction You may have heard that light consists of particles called photons. How could something as simple as light be made of particles? Physicists describe light as both a particle and a wave. In fact, light's wavelike behavior is responsible for a lot of its cool effects, such as the iridescent colors produced on the surface of bubbles. To see a dramatic and mind-bending example of how light behaves like a wave, all you need is three pieces of mechanical pencil lead, a laser pointer and a dark room.
Background Sound is a great example of a wave that propagates, or travels, much like ripples in a pond do. In both cases kinetic energy flows through matter without permanently displacing the molecules in the matter itself—instead, it puts the matter through phases of compression (where the molecules get pushed together) and rarefaction (where the molecules spread apart). Think of the inside of a speaker vibrating with the music.
On supporting science journalism
If you're enjoying this article, consider supporting our award-winning journalism by subscribing . By purchasing a subscription you are helping to ensure the future of impactful stories about the discoveries and ideas shaping our world today.
When waves come into contact with one another, they exhibit interference: waves that are all in phase (rarefying or compressing the same particles at the same time) add together to become stronger, and waves that are out of phase with one another (for example, one wave attempts to rarefy particles in a medium while another attempts to compress those same particles) cancel out. This is how noise-canceling headphones work—they produce a sound wave that resembles the wave responsible for the unwanted sound, but with the original phases of rarefaction and compression flipped. This has the effect of dampening the offending sound wave's effect on the air molecules. So that by the time its energy reaches your ear, the sound you perceive is more of a whisper than a shout—or an airplane engine's roar is more like a quiet hum.
Diffraction is another important feature of waves: When waves encounter small openings, they spread out after they pass through. In the following experiment we'll set up two slits to give waves of light the opportunity to diffract as they travel through them. The different points at which the diffracted waves overlap should demonstrate some cool patterns of constructive and destructive interference, and you'll get to witness the puzzling effect of light "canceling itself out."
Materials • Three or more pieces of mechanical pencil lead (either 0.5 or 0.7 millimeter) • Laser pointer (Red will work just fine, but green produces a more dramatic effect.) • Dark room
Procedure • Darken the room. • Stand about four feet from a wall. • Hold the three pieces of pencil lead between your thumb and forefinger of your nondominant hand (your left hand if you are right-handed). Spread them apart very slightly so that you create two tiny gaps between the leads. These will act as your diffraction slits. • Shine your laser through the slits you created, and look at the pattern of light emitted on the wall. What do you see? • Play around with the positioning of your pencil leads, your laser and the width of the slits you created. When you get everything right, you should see a distinct pattern of dots appear on the wall behind the pencil lead. • Extra : Try using more pencil leads to create more diffraction slits. How does adding more slits change the pattern of light projected on the wall? Results and observations Laser light is emitted in the form of parallel waves that are coherent, or in phase with one another: all of the peaks and valleys line up. This is quite different than the light emitted from a flashlight—the rays are neither parallel nor in phase with each other. The laser's waves diffract as they pass through the slits you made, fanning out in a shotgunlike pattern from each slit. This allows them to interfere with one another as they overlap. In some places the waves will interfere constructively, creating bright spots on the wall. In other places the waves cancel themselves out, leading to the dark spaces you see between the spots.
If light demonstrated particlelike behavior exclusively, you would see only two dots on the wall corresponding to the locations of the slits. Oddly enough, Isaac Newton understood light this way: as a stream of particles, like a series of baseballs being thrown in a straight line. The problem posed by the double-slit experiment is that "baseballs" thrown through one hole seem to care about what the baseballs thrown through the other hole do! In the 19th century scientists decided that light must be a wave, but after witnessing light demonstrating particlelike behavior, Albert Einstein proposed that light can indeed be described as a particle (called a photon). The physicist Max Planck panicked, claiming, "the theory of light would be thrown back not by decades, but by centuries" if the scientific community were to accept Einstein's theory! But scientists ultimately arrived at the conclusion that light is both a particle (photon) and a wave.
Think of light's wave function as corresponding to the likelihood of a photon being in a certain place at a certain time. This makes it a little easier to understand how photons are forced to arrive at certain positions on the wall when their waves interfere with one another. What's less intuitive is the fact that photons fired one at a time toward two slits still demonstrate the same wavelike interference behavior after they pass through—it's as though individual photons are able to travel through two slits at once while still arriving at one location!
More to Explore The Original Double-Slit Experiment , from Veritasium Double-Slit Experiment Explained! By Jim Al-Khalili , from The Royal Institution Wave–Particle Duality of Light , from Education.com Young's Double-Slit Experiment , from YouTube Bubble Colors , from The Exploratorium
This activity brought to you in partnership with Education.com
Thank you for visiting nature.com. You are using a browser version with limited support for CSS. To obtain the best experience, we recommend you use a more up to date browser (or turn off compatibility mode in Internet Explorer). In the meantime, to ensure continued support, we are displaying the site without styles and JavaScript.
- View all journals
- Explore content
- About the journal
- Publish with us
- Sign up for alerts
- 13 June 2023
- Correction 20 June 2023
Particle, wave, both or neither? The experiment that challenges all we know about reality
- Anil Ananthaswamy 0
Anil Ananthaswamy is a writer based in California, and author of Through Two Doors at Once .
You can also search for this author in PubMed Google Scholar
You have full access to this article via your institution.
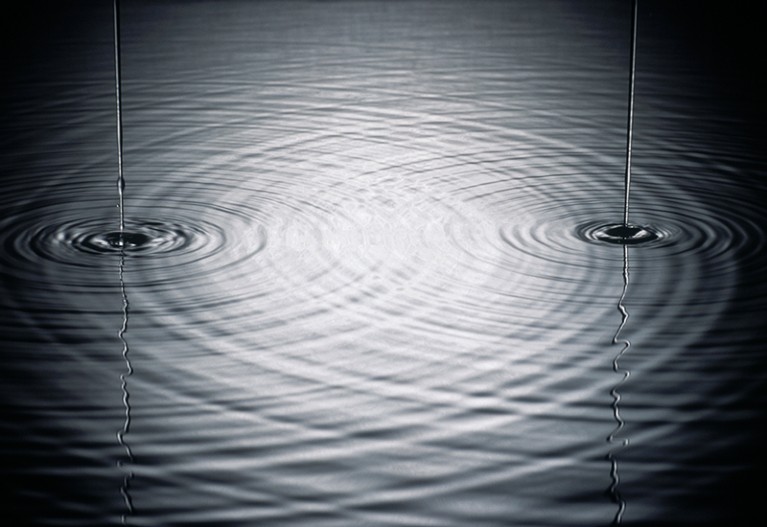
The double-slit experiment’s interference patterns suggest something is in two places at once. Credit: Huw Jones/Getty
Thomas Young, born 250 years ago this week, was a polymath who made seminal contributions in fields from physics to Egyptology . But perhaps his most enduring legacy is proving Isaac Newton wrong about light — and igniting a debate about the nature of reality that still persists.
“The experiments I am about to relate”, he told the Royal Society of London 1 on 24 November 1803, “may be repeated with great ease, whenever the sun shines.” In a simple, modern form, Young’s ‘double-slit’ experiment involves shining light of a single frequency (say, from a red laser) through two fine, parallel openings in an opaque sheet, onto a screen beyond. If light were made of streams of particles, as Newton conjectured, you would expect to see two distinct strips of light on the screen, where the particles pile up after travelling through one slit or the other. But that’s not what happens. Instead, you see many bands of light and dark, strung out in stripes like a barcode: an interference pattern (see ‘Wave–particle weirdness’).
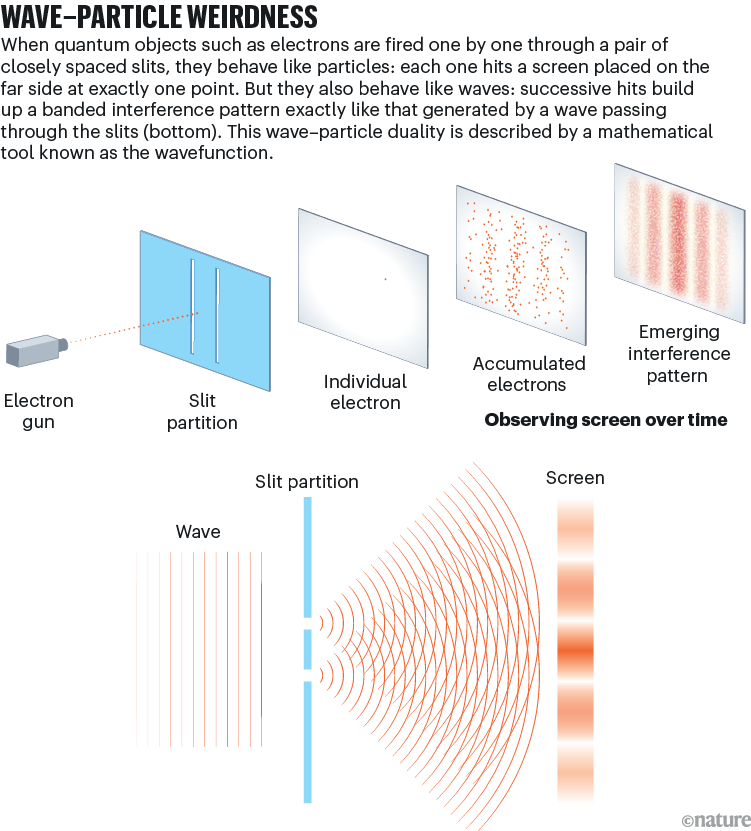
Interference is possible only if light behaves as a wave that strikes both slits at once and diffracts through each, creating two sets of waves on the other side of the slits that propagate towards the screen. Where the crest of one wave overlaps with the crest of the other, you get constructive interference and a patch of light. Where a crest meets a trough, you get destructive interference and darkness.
It’s hard to overstate how wild this discovery was to physicists in Young’s time. But the wildness truly began when Max Planck and Albert Einstein laid the foundations for quantum mechanics in the early twentieth century. Today, quantum mechanics forms a peerlessly accurate framework to explain the basic elements of material reality and their interactions. Pretty early on, it became clear that it implied that light is made of indivisible units of energy called photons — particles, in fact. The amount of energy each carried was proportional to the frequency of the light. Some carry enough of a wallop to knock electrons off atoms of metal, giving us the photoelectric effect that enables today’s solar cells. (It was the study of this effect that led Einstein to his conclusions about light’s particulate nature.)
With the emergence of quantum mechanics, the idea of light as a wave faced a challenge. But it wasn’t as simple as going back to the particle view. Further tests of quantum theory using the double-slit experiment only deepened the mystery. And it hasn’t been solved yet.
Singularly quantum
Imagine, now, that your light source can shoot individual photons of red light at the two slits, while guaranteeing that only one photon goes through the apparatus at any time. A photographic plate on the other side records where the photons land. Classical intuition says each photon can go through only one slit or the other. So, this time, we should see photons accumulating over time and forming two strips of light on the photographic plate. Yet the mathematics of quantum theory implied that the interference pattern would persist.
It was several decades before the technology matured enough to verify these predictions experimentally, using more complex set-ups that were in principle the double-slit. At first, it wasn’t done with photons, but with electrons — entities that we know as particles, but that quantum mechanics predicts act as waves, too. Then, in the 1980s, a team led by Alain Aspect at the Optical Institute in Palaiseau, France, performed the double-slit experiment with single photons 2 . Quantum theory won out: an interference pattern emerged, even when only single particles passed through the slits.
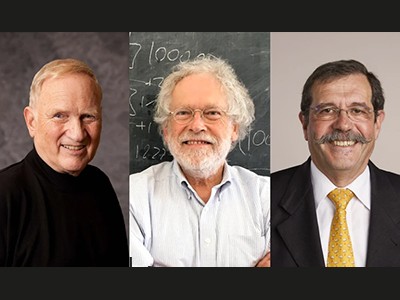
‘Spooky’ quantum-entanglement experiments win physics Nobel
Aspect won a share of the 2022 Nobel prize in physics for his contribution to confirming the predictions of quantum mechanics through experiment. But such experiments leave matters of interpretation wide open. There is simply no way to comprehend what’s happening with minds attuned to the classical world of everyday objects.
When it comes to the double-slit experiment, quantum mechanics does tell a form of story. It says that a photon’s position is described by a mathematical abstraction called the wavefunction — which, as the name suggests, behaves like a wave. This wavefunction, mathematically speaking, hits the two slits, diffracts into two sets of waves and recombines to create the interference pattern. The value of the wavefunction at any location on the photographic plate lets you calculate the probability of finding the photon there. The probability is very high in regions of constructive interference, and very low in regions of destructive interference.
In a sense, then, a photon or any other quantum object acts like both a particle and a wave. This ‘wave–particle duality’ embodies many of the central conceptual mysteries of quantum mechanics that are unresolved to this day. Even if you could know everything about a photon’s initial state, there’s no way to tell exactly where it’ll land on the detector. You have to talk in terms of probabilities given by the wavefunction. These probabilities are borne out only when thousands or tens of thousands of photons are sent through the double slit, one by one.
Before the measurement — in this case, detection by the photographic plate — the mathematics says the particle exists in a superposition of states: in a sense, it has taken both paths, through the right slit and the left. Standard quantum mechanics says that the wavefunction ‘collapses’ when measured, and that the act of observation in some way precipitates that collapse. Before this, the photon has a finite probability of being found in many different regions, but on measurement, the wavefunction peaks at the location in which the photon appears (the probability there equals 1) and is nullified everywhere else (probability equals 0).
It gets even odder. If you can determine which path the photon took on its way to the detector, it acts like a particle that does indeed go through one slit or the other: the interference pattern disappears. But if you cannot glean this ‘which-way’ information, the photon acts like a wave. Whenever there are two or more ways for a photon — or, indeed, any quantum object — to get to a final state, quantum interference occurs.
What’s a wavefunction?
But to generate interference, something has to go through — or at least interact in some way with — both slits. In the mathematics, the wavefunction does the job. Some physicists would say that the wavefunction simply represents information about the quantum system and is not real — in which case it’s hard to explain what interacts with both slits at once. But you can explain the interference pattern if you consider the wavefunction to be real.
This creates its own problems. Imagine a real wavefunction that spreads for kilometres and kilometres before an observer detects the photon. At this point, the wavefunction peaks at the photon’s location, and simultaneously drops to zero everywhere else — over a large, macroscopic distance. This suggests a kind of instantaneous, non-local influence that bothered Einstein no end. One can avoid this with interpretations of quantum theory that don’t collapse the wavefunction, but that opens other cans of worms.
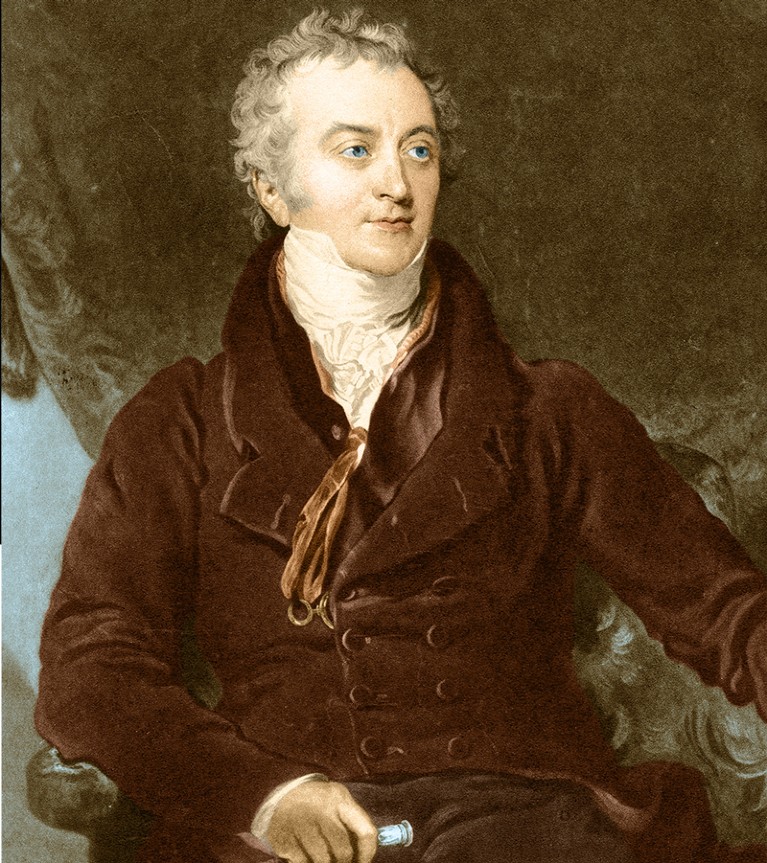
Thomas Young reported the results of the original double-slit experiment in the early nineteenth century. Credit: Photo Researchers/SPL
Perhaps the most notorious is the many-worlds interpretation, the brainchild of US physicist Hugh Everett in the 1950s. This argues that every possible event — in the case of the double slit, a particle going through the left and the right slit — happens, each in its own world. There is no collapse: measurement simply reveals the state of the quantum system in that world. Detractors ask how it’s possible to justify this constant proliferation of worlds, and how, in a many-worlds framework, you can explain why measuring quantum systems yields probabilities, given that there are always definite outcomes in each world.
The de Broglie–Bohm theory, named after quantum pioneers Louis de Broglie and David Bohm, provides another alternative. It says that particles exist with definite positions and momenta, but are guided by an all-encompassing, invisible ‘pilot’ wave, and it’s this wave that goes through both slits. The most profound implication of this theory, that everything is linked to everything else in the Universe by the underlying pilot wave, is one many physicists have trouble accepting.
In the 1970s and 1980s, physicists upgraded the double-slit experiment to seek clarity about the nature of quantum reality, and the perplexing role observation apparently has in collapsing a defined, classical reality out of it. Most notably, John Wheeler at the University of Texas at Austin designed the ‘delayed choice’ thought experiment 3 . Imagine a double-slit set-up that gives the option of gathering or ignoring information about which way the particle went. If you ignore the ‘which-way’ information, you get wave-like behaviour; if you don’t, you get particle-like patterns.
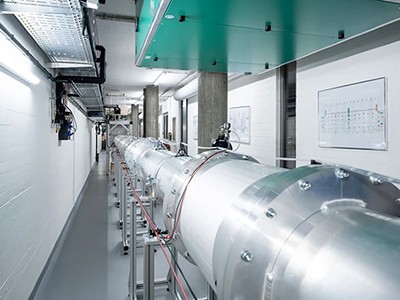
Superconducting qubits cover new distances
With the apparatus on the ‘collect which-way information’ setting, send a photon through the double slits. It should act like a particle and go through one slit or the other. But just before the photon lands on the detector, flip the apparatus to ignore the which-way information. Will the photon, until then supposedly a particle, suddenly switch to being a wave?
Decades later, Aspect’s team performed this experiment with single photons and showed that the answer is yes 4 . Even if the photon had ostensibly travelled through the entire set-up as a particle, switching the apparatus setting so that it ignored which-way information caused it to act like a wave. Did the photon travel back in time and come back through the two slits as a wave? To avoid such nonsensical explanations, Wheeler argued that the only way to make sense of the experiment was to say that the photon has no reality — it’s neither wave nor particle — until it’s detected.
Back in the 1980s, Marlan Scully, then at the University of New Mexico in Albuquerque, and his colleagues came up with a similarly befuddling thought experiment 5 . They imagined collecting the which-way information about a photon by using a second photon ‘entangled’ with the first — a situation in which measuring the quantum state of one tells you about the quantum state of the other. As long as the which-way information can in principle be extracted, the first photon should act like a particle. But if you erase the information in the entangled partner, the mathematics showed, the first photon goes back to behaving like a wave. In 2000, Scully, Yoon-Ho Kim and their colleagues reported performing this experiment 6 . Surprisingly — or unsurprisingly, by this stage — intuition was once again defeated and quantum weirdness reigned supreme.
Larger and still larger
Others are still pushing the double slit in new directions. This year, Romain Tirole at Imperial College London and his colleagues described an experiment in which the slits were temporal: one slit was open at one point in time and the second slit an instant later 7 . A beam of light that goes through these temporal slits produces an interference pattern in its frequency spectrum. Again, the mathematics predicts exactly this behaviour, so physicists aren’t surprised. But it is more proof that the double-slit experiment highlights the lacunae in our understanding of reality, a quarter of a millennium after the birth of the man who devised it.
The double-slit experiment’s place in the pantheon of physics experiments is assured. But it would be further cemented if and when physicists using it were able to work out which theory of the quantum world is correct.
For example, some theories posit that quantum systems that grow bigger than a certain, as-yet-undetermined size randomly collapse into classical systems, with no observer needed. This would explain why macroscopic objects around us don’t obviously work according to quantum rules — but how big does something have to be before it stops acting in a quantum way?
In 2019, Markus Arndt and Yaakov Fein at the University of Vienna and their colleagues reported sending macromolecules called oligoporphyrins, composed of up to 2,000 atoms, through a double slit to see whether they produce an interference pattern 8 . They do, and these patterns can be explained only as a quantum phenomenon. Arndt’s team and others continue to push such experiments to determine whether a line exists between the quantum and the classical world.
Last year, Siddhant Das at the Ludwig Maximilian University of Munich, Germany, and his colleagues analysed the double-slit experiment in the context of the de Broglie–Bohm theory 9 . Unlike standard quantum mechanics, this predicts not just the distribution of particles on the screen that leads to the spatial interference pattern, but also the distribution of when the particles arrive at the screen. The researchers found that their calculations on the distribution of arrival times agreed qualitatively with observations made two decades before, in a double-slit experiment using helium atoms 10 . But it was difficult to prove their case definitively. They are awaiting better data from a similar double-slit experiment done with current technology, to see whether it matches predictions.
And so it goes on, a world away from anything Young or his peers at the Royal Society could have conceived of more than two centuries ago. “Thomas Young would probably scratch his head if he could see the status of today’s experiments,” says Arndt. But that’s because his experiment, so simple in concept, has left us scratching our heads to this day.
Nature 618 , 454-456 (2023)
doi: https://doi.org/10.1038/d41586-023-01938-6
Updates & Corrections
Correction 20 June 2023 : An earlier version of the second picture caption gave the wrong date for when Young reported results of a double-slit experiment.
Young, T. Phil. Trans. R. Soc. 94 , 1–16 (1804).
Google Scholar
Grangier, P., Roger, G. & Aspect, A. Europhys. Lett. 1 , 173 (1986).
Article Google Scholar
Miller, W. A. & Wheeler, J. A. Foundations of Quantum Mechanics in the Light of New Technology (Eds Nakajima, S., Murayama, Y. & Tonomura, A.) 72–84 (World Scientific, 1997).
Jacques, V. et al. Science 315 , 966–968 (2007).
Article PubMed Google Scholar
Scully, M. O. & Drühl, K. Phys. Rev. A. 25 , 2208 (1982).
Kim, Y.-H. et al. Phys. Rev. Lett. 84 , 1 (2000).
Tirole, R. et al. Nature Phys . https://doi.org/10.1038/s41567-023-01993-w (2023).
Fein, Y. Y. et al. Nature Phys. 15 , 1242–1245 (2019).
Das, S., Deckert, D.-A., Kellers, L. & Struyve, W. Preprint at https://arxiv.org/abs/2211.13362 (2022).
Kurtsiefer, Ch., Pfau T. & Mlynek, J. Nature 386 , 150–153 (1997).
Download references
Competing Interests
The author declares no competing interests.
Related Articles
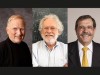
- Quantum physics
- Quantum information
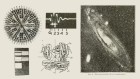
Brunch with a carnivorous plant
News & Views 03 SEP 24
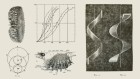
Man versus horse: who wins?
News & Views 27 AUG 24
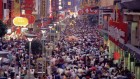
The meaning of the Anthropocene: why it matters even without a formal geological definition
Comment 26 AUG 24
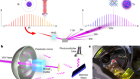
Frequency ratio of the 229mTh nuclear isomeric transition and the 87Sr atomic clock
Article 04 SEP 24
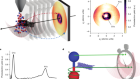
Attosecond delays in X-ray molecular ionization
Article 21 AUG 24
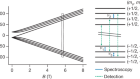
Precision spectroscopy on 9Be overcomes limitations from nuclear structure
Article 14 AUG 24
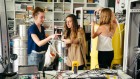
Quantum computing aims for diversity, one qubit at a time
Technology Feature 05 AUG 24
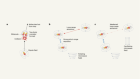
Ultracold molecules that interact from afar form elusive quantum state
News & Views 09 JUL 24
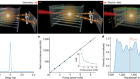
Tunable entangled photon-pair generation in a liquid crystal
Article 12 JUN 24
Postdoctoral Associate- Environmental Epidemiology
Houston, Texas (US)
Baylor College of Medicine (BCM)
Open Faculty Positions at the State Key Laboratory of Brain Cognition & Brain-inspired Intelligence
The laboratory focuses on understanding the mechanisms of brain intelligence and developing the theory and techniques of brain-inspired intelligence.
Shanghai, China
CAS Center for Excellence in Brain Science and Intelligence Technology (CEBSIT)
Research Associate - Good Manufacturing Practices (GMP)
Faculty position in pathology research.
Dallas, Texas (US)
The University of Texas Southwestern Medical Center (UT Southwestern Medical Center)
Director, Clinical Translational Program, Center for Alzheimer’s and Related Dementias
The Intramural Research Programs (IRPs) of the National Institute on Aging (NIA)
Bethesda, Maryland (US)
National Institutes of Health
Sign up for the Nature Briefing newsletter — what matters in science, free to your inbox daily.
Quick links
- Explore articles by subject
- Guide to authors
- Editorial policies

Advertisement
How Light Works
- Share Content on Facebook
- Share Content on LinkedIn
- Share Content on Flipboard
- Share Content on Reddit
- Share Content via Email
Light as Waves
Unlike water waves, light waves follow more complicated paths, and they don't need a medium to travel through.
When the 19th century dawned, no real evidence had accumulated to prove the wave theory of light. That changed in 1801 when Thomas Young, an English physician and physicist, designed and ran one of the most famous experiments in the history of science. It's known today as the double-slit experiment and requires simple equipment -- a light source, a thin card with two holes cut side by side and a screen.
To run the experiment, Young allowed a beam of light to pass through a pinhole and strike the card. If light contained particles or simple straight-line rays, he reasoned, light not blocked by the opaque card would pass through the slits and travel in a straight line to the screen, where it would form two bright spots. This isn't what Young observed. Instead, he saw a bar code pattern of alternating light and dark bands on the screen. To explain this unexpected pattern, he imagined light traveling through space like a water wave, with crests and troughs. Thinking this way, he concluded that light waves traveled through each of the slits, creating two separate wave fronts. As these wave fronts arrived at the screen, they interfered with each other. Bright bands formed where two wave crests overlapped and added together. Dark bands formed where crests and troughs lined up and canceled each other out completely.
Young's work sparked a new way of thinking about light. Scientists began referring to light waves and reshaped their descriptions of reflection and refraction accordingly, noting that light waves still obey the laws of reflection and refraction. Incidentally, the bending of a light wave accounts for some of the visual phenomena we often encounter, such as mirages . A mirage is an optical illusion caused when light waves moving from the sky toward the ground are bent by the heated air.
In the 1860s, Scottish physicist James Clerk Maxwell put the cherry on top of the light-wave model when he formulated the theory of electromagnetism . Maxwell described light as a very special kind of wave -- one composed of electric and magnetic fields. The fields vibrate at right angles to the direction of movement of the wave, and at right angles to each other. Because light has both electric and magnetic fields, it's also referred to as electromagnetic radiation . Electromagnetic radiation doesn't need a medium to travel through, and, when it's traveling in a vacuum, moves at 186,000 miles per second (300,000 kilometers per second). Scientists refer to this as the speed of light , one of the most important numbers in physics.
Please copy/paste the following text to properly cite this HowStuffWorks.com article:

- Why Does Water Expand When It Freezes
- Gold Foil Experiment
- Faraday Cage
- Oil Drop Experiment
- Magnetic Monopole
- Why Do Fireflies Light Up
- Types of Blood Cells With Their Structure, and Functions
- The Main Parts of a Plant With Their Functions
- Parts of a Flower With Their Structure and Functions
- Parts of a Leaf With Their Structure and Functions
- Why Does Ice Float on Water
- Why Does Oil Float on Water
- How Do Clouds Form
- What Causes Lightning
- How are Diamonds Made
- Types of Meteorites
- Types of Volcanoes
- Types of Rocks
Double Slit Experiment
What is the double-slit experiment.
The double-slit experiment is a test that demonstrates light can fundamentally display both wave and particle features, also known as wave-particle (photon) duality. It consists of two closely-spaced slits in front of a light source such that a beam of light passing through them is split and projected on a screen placed far from the slits. The split beam forms two coherent sources of light. A pattern of bright and dark fringes appears on the screen due to the interference of light waves coming from these two sources. Interference is the phenomenon in which when waves are superimposed, they may reinforce or cancel each other out. It is most pronounced when the wavelength of the radiation is comparable to the linear dimensions of the slits.
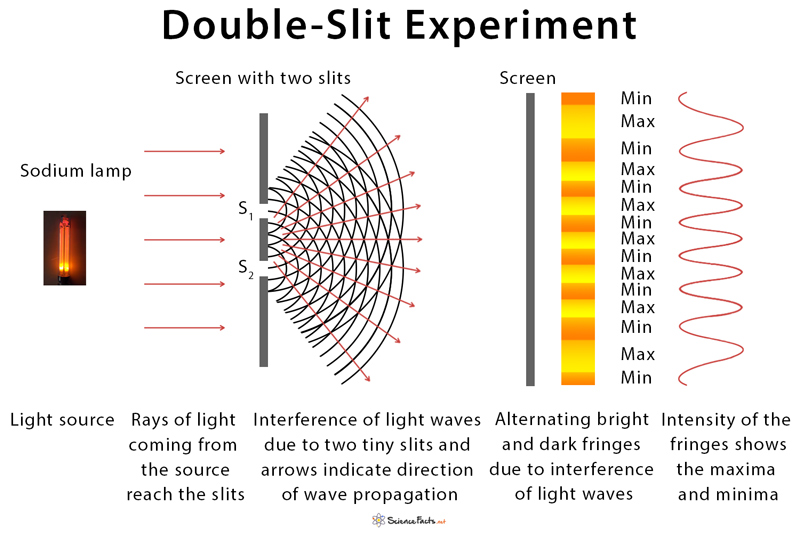
The history of this experiment goes back to 1801 when British polymath Thomas Young demonstrated the wave nature of light and hence, is often called Young’s double-slit experiment.
Double-Slit Experiment Equation
A monochromatic light source falls on a screen that contains two parallel slits, which serve as the sources of coherent light. The light waves emerging from the two slits then interfere and form an interference pattern on the viewing screen. The bright fringes or bands correspond to interference maxima, and the dark fringes are interference minima.
To locate the fringes from the center of the screen, one assumes that the distance between the screen and slit is much greater than the distance separating the slits. Also, the separation between the slits is much greater than the wavelength of the monochromatic light.
By measuring the separation between the fringes and knowing the distance of the screen from the slits and distance between the two slits, it is possible to determine the wavelength of light.
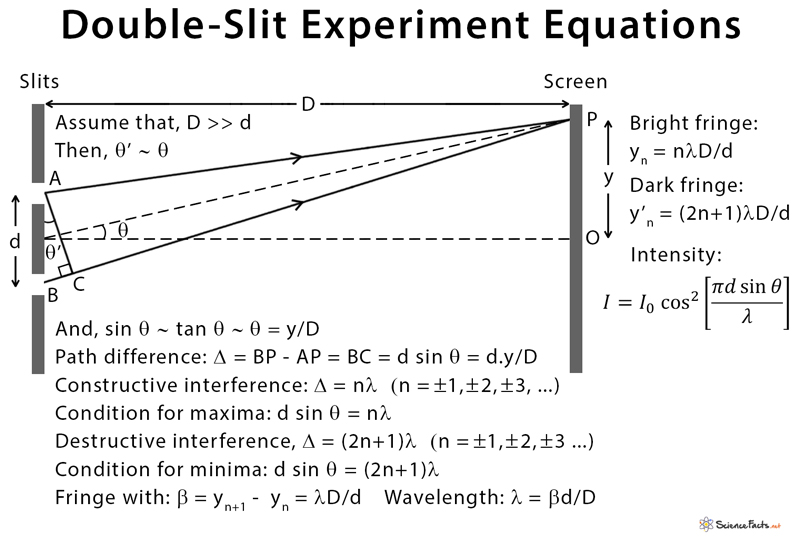
Ans. The double-slit experiment was a groundbreaking test that decisively proves the theory that light behaves as a wave.
Ans . Yes. Many researchers have performed the double-slit experiment on electrons and found similar results to that of photons.
Article was last reviewed on Wednesday, June 3, 2020
Related articles

Leave a Reply Cancel reply
Your email address will not be published. Required fields are marked *
Save my name, email, and website in this browser for the next time I comment.
Popular Articles
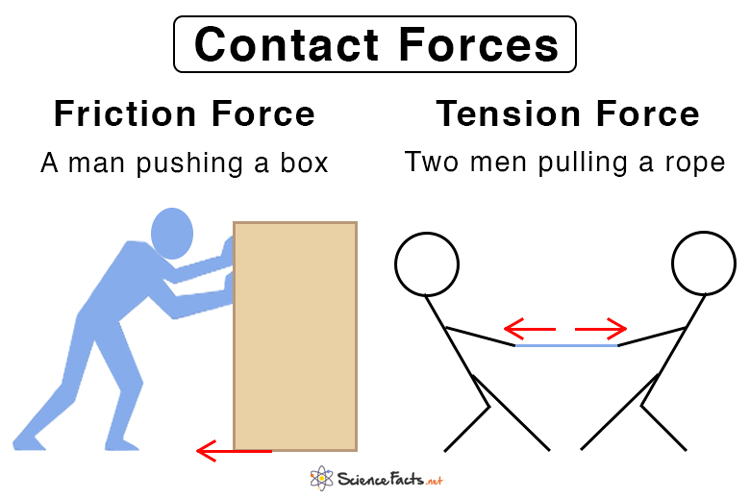
Join our Newsletter
Fill your E-mail Address
Related Worksheets
- Privacy Policy
© 2024 ( Science Facts ). All rights reserved. Reproduction in whole or in part without permission is prohibited.
Double-slit experiment that proved the wave nature of light explored in time
by Thomas Angus [Photographer] , Hayley Dunning 03 April 2023
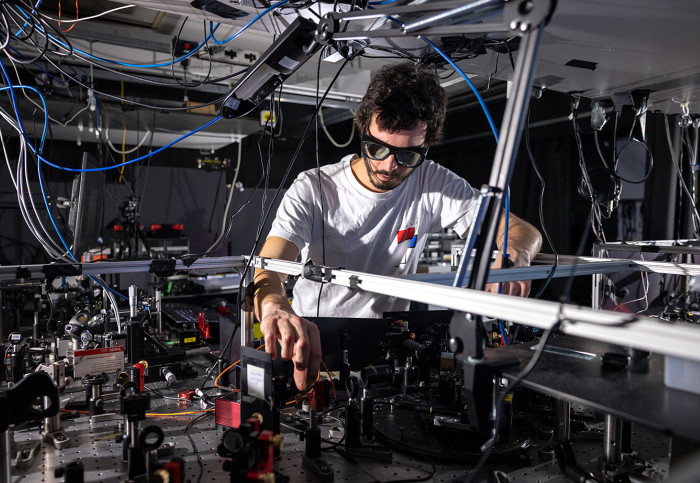
Imperial physicists have recreated the famous double-slit experiment, which showed light behaving as particles and a wave, in time rather than space.
The experiment relies on materials that can change their optical properties in fractions of a second, which could be used in new technologies or to explore fundamental questions in physics.
Our experiment reveals more about the fundamental nature of light while serving as a stepping-stone to creating the ultimate materials that can minutely control light in both space and time. Professor Riccardo Sapienza
The original double-slit experiment, performed in 1801 by Thomas Young at the Royal Institution, showed that light acts as a wave. Further experiments, however, showed that light actually behaves as both a wave and as particles – revealing its quantum nature.
These experiments had a profound impact on quantum physics, revealing the dual particle and wave nature of not just light, but other ‘particles’ including electrons, neutrons, and whole atoms.
Now, a team led by Imperial College London physicists has performed the experiment using ‘slits’ in time rather than space. They achieved this by firing light through a material that changes its properties in femtoseconds (quadrillionths of a second), only allowing light to pass through at specific times in quick succession.
Lead researcher Professor Riccardo Sapienza , from the Department of Physics at Imperial, said: “Our experiment reveals more about the fundamental nature of light while serving as a stepping-stone to creating the ultimate materials that can minutely control light in both space and time.”
Details of the experiment are published today in Nature Physics .
Patterns of interference
The original double-slit setup involved directing light at an opaque screen with two thin parallel slits in it. Behind the screen was a detector for the light that passed through.
To travel through the slits as a wave, light splits into two waves that go through each slit. When these waves cross over again on the other side, they ‘interfere’ with each other. Where peaks of the wave meet, they enhance each other, but where a peak and a trough meet, they cancel each other out. This creates a striped pattern on the detector of regions of more light and less light.
Light can also be parcelled up into ‘particles’ called photons, which can be recorded hitting the detector one at a time, gradually building up the striped interference pattern. Even when researchers fired just one photon at a time, the interference pattern still emerged, as if the photon split in two and travelled through both slits.
In the classic version of the experiment, light emerging from the physical slits changes its direction, so the interference pattern is written in the angular profile of the light.
Instead, the time slits in the new experiment change the frequency of the light, which alters its colour. This created colours of light that interfere with each other, enhancing and cancelling out certain colours to produce an interference-type pattern.
Metamaterials and time crystals
The material the team used was a thin film of indium-tin-oxide, which forms most mobile phone screens. The material had its reflectance changed by lasers on ultrafast timescales, creating the ‘slits’ for light. The material responded much quicker than the team expected to the laser control, varying its reflectivity in a few femtoseconds.
The material is a metamaterial – one that is engineered to have properties not found in nature. Such fine control of light is one of the promises of metamaterials, and when coupled with spatial control, could create new technologies and even analogues for studying fundamental physics phenomena like black holes.
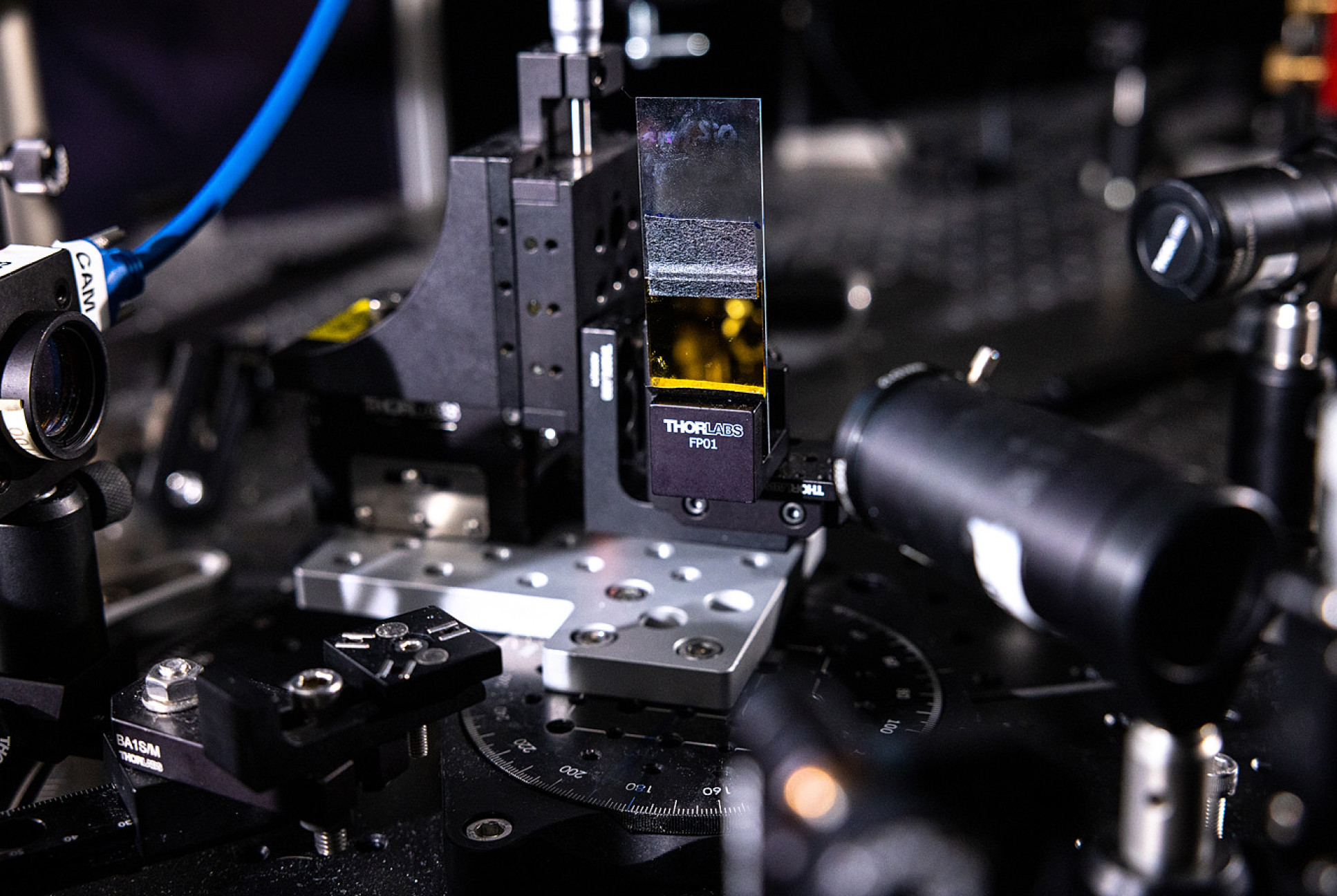
Co-author Professor Sir John Pendry said: "The double time slits experiment opens the door to a whole new spectroscopy capable of resolving the temporal structure of a light pulse on the scale of one period of the radiation."
The team next want to explore the phenomenon in a ‘time crystal’, which is analogous to an atomic crystal, but where the optical properties vary in time.
Co-author Professor Stefan Maier said: “The concept of time crystals has the potential to lead to ultrafast, parallelized optical switches.”
‘ Double-slit time diffraction at optical frequencies ’ by Romain Tirole, Stefano Vezzoli, Emanuele Galiffi, Iain Robertson, Dries Maurice, Benjamin Tilmann, Stefan A. Maier, John B. Pendry and Riccardo Sapienza is published in Nature Physics .
Article text (excluding photos or graphics) © Imperial College London.
Photos and graphics subject to third party copyright used with permission or © Imperial College London.
Thomas Angus [Photographer] Communications Division

Contact details
Tel: +44 (0)20 7594 2858 Email: [email protected] Show all stories by this author
Hayley Dunning Communications Division
Tel: +44 (0)20 7594 2412 Email: [email protected] Show all stories by this author
Research , Materials , REF See more tags
Comments are loading...
Leave a comment
Your comment may be published, displaying your name as you provide it, unless you request otherwise. Your contact details will never be published.
Latest news

Science leader
Imperial professor appointed executive chair of major uk science funding body, major european grants, imperial engineers win european grants for turbulence and computational sensing, immune insights, sex hormones modulate the immune system to influence disease risk differently, most popular, 1 science leader:, 2 infection study:, covid-19 – how long am i infectious and when can i safely leave isolation, 3 psychedelic treatment:, magic mushroom compound increases brain connectivity in people with depression, 4 immune insights:, latest comments, comment on professor david q mayne freng frs 1930 - 2024 : i am deeply saddened to learn of professor mayne’s passing. he was a kind and decent man who super…, comment on professor david q mayne freng frs 1930 - 2024 : so sad to know that a great man has passed on. may david mayne's soul rest in peace. i quite remembe…, comment on professor david q mayne freng frs 1930 - 2024 : i worked as secretary for professor westcott in the control systems group in the late 1960s and reme…, latest tweets.
share this!
March 2, 2015
The first ever photograph of light as both a particle and wave
by Ecole Polytechnique Federale de Lausanne
(Phys.org)—Light behaves both as a particle and as a wave. Since the days of Einstein, scientists have been trying to directly observe both of these aspects of light at the same time. Now, scientists at EPFL have succeeded in capturing the first-ever snapshot of this dual behavior.
Quantum mechanics tells us that light can behave simultaneously as a particle or a wave. However, there has never been an experiment able to capture both natures of light at the same time; the closest we have come is seeing either wave or particle, but always at different times. Taking a radically different experimental approach, EPFL scientists have now been able to take the first ever snapshot of light behaving both as a wave and as a particle. The breakthrough work is published in Nature Communications .
When UV light hits a metal surface, it causes an emission of electrons . Albert Einstein explained this "photoelectric" effect by proposing that light – thought to only be a wave – is also a stream of particles. Even though a variety of experiments have successfully observed both the particle- and wave-like behaviors of light, they have never been able to observe both at the same time.
A research team led by Fabrizio Carbone at EPFL has now carried out an experiment with a clever twist: using electrons to image light. The researchers have captured, for the first time ever, a single snapshot of light behaving simultaneously as both a wave and a stream of particles.
The experiment is set up like this: A pulse of laser light is fired at a tiny metallic nanowire. The laser adds energy to the charged particles in the nanowire, causing them to vibrate. Light travels along this tiny wire in two possible directions, like cars on a highway. When waves traveling in opposite directions meet each other they form a new wave that looks like it is standing in place. Here, this standing wave becomes the source of light for the experiment, radiating around the nanowire.
This is where the experiment's trick comes in: The scientists shot a stream of electrons close to the nanowire, using them to image the standing wave of light. As the electrons interacted with the confined light on the nanowire, they either sped up or slowed down. Using the ultrafast microscope to image the position where this change in speed occurred, Carbone's team could now visualize the standing wave, which acts as a fingerprint of the wave-nature of light.
While this phenomenon shows the wave-like nature of light, it simultaneously demonstrated its particle aspect as well. As the electrons pass close to the standing wave of light, they "hit" the light's particles , the photons. As mentioned above, this affects their speed, making them move faster or slower. This change in speed appears as an exchange of energy "packets" (quanta) between electrons and photons. The very occurrence of these energy packets shows that the light on the nanowire behaves as a particle.
"This experiment demonstrates that, for the first time ever, we can film quantum mechanics – and its paradoxical nature – directly," says Fabrizio Carbone. In addition, the importance of this pioneering work can extend beyond fundamental science and to future technologies. As Carbone explains: "Being able to image and control quantum phenomena at the nanometer scale like this opens up a new route towards quantum computing."
Journal information: Nature Communications
Provided by Ecole Polytechnique Federale de Lausanne
Explore further
Feedback to editors

'Some pterosaurs would flap, others would soar'—new study confirms flight capability of these giants of the skies
28 minutes ago

AI meets biophysics: New approach identifies critical interaction points in cancer-related proteins
7 hours ago

Study shows how amateur astronomers can aid in Jupiter weather monitoring

Guardians of the reef: How parrotfish promote coral health
8 hours ago

New mRNA and gene editing tools offer hope for dengue virus treatment

Researchers examine how drought and water volume affect nutrients in Apalachicola river

Gravitational waves unveil previously unseen properties of neutron stars

Space-based experiments could help to advance early cancer detection through blood tests

Phage editing technology could lead to alternative treatments for antibiotic-resistant bacteria

Decoding the language of cells with the power of proteomics
Relevant physicsforums posts, why tilting a diffraction grating produces tilted dots.
Sep 2, 2024
Spherical Lens Model and Proof
Interpretation of a complex angle of refraction.
Aug 28, 2024
The double-slit experiment with a pit in the screen
Aug 19, 2024
How to observe difference of L/R rotation intensity of CPL
Jul 29, 2024
Increase in numerical aperture leads to a decrease in line width?
Jul 19, 2024
More from Optics
Related Stories

Optical 'watermills' control spinning light
Nov 14, 2014
'One real mystery of quantum mechanics': Physicists devise new experiment
Nov 1, 2012

Quantum physics just got less complicated
Dec 19, 2014

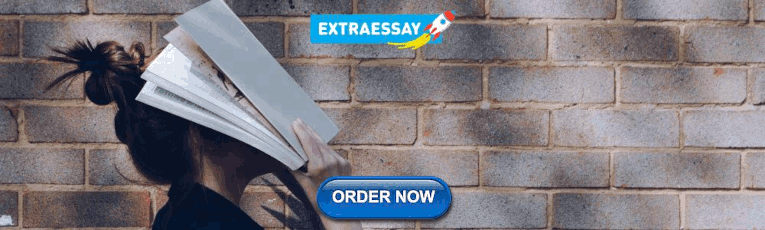
New analysis shows a way to self-propel subatomic particles
Jan 21, 2015

Quantum computer as detector shows space is not squeezed
Jan 28, 2015

Could classical theory be just as weird as quantum theory?
Feb 23, 2015
Recommended for you

A window into the body: New technique makes skin invisible
10 hours ago

Novel metasurface enables temperature-adaptive radiative cooling
20 hours ago

Optoelectronic diamond device reveals an unexpected phenomenon reminiscent of lightning in slow motion
Sep 4, 2024

Entangled photon pairs enable hidden image encoding

The world's fastest single-shot 2D imaging technique films ultrafast dynamics in flames
Sep 3, 2024

Researchers demonstrate spontaneous synchronization of quantum vortices in semiconductor microcavities
Let us know if there is a problem with our content.
Use this form if you have come across a typo, inaccuracy or would like to send an edit request for the content on this page. For general inquiries, please use our contact form . For general feedback, use the public comments section below (please adhere to guidelines ).
Please select the most appropriate category to facilitate processing of your request
Thank you for taking time to provide your feedback to the editors.
Your feedback is important to us. However, we do not guarantee individual replies due to the high volume of messages.
E-mail the story
Your email address is used only to let the recipient know who sent the email. Neither your address nor the recipient's address will be used for any other purpose. The information you enter will appear in your e-mail message and is not retained by Phys.org in any form.
Newsletter sign up
Get weekly and/or daily updates delivered to your inbox. You can unsubscribe at any time and we'll never share your details to third parties.
More information Privacy policy
Donate and enjoy an ad-free experience
We keep our content available to everyone. Consider supporting Science X's mission by getting a premium account.
E-mail newsletter
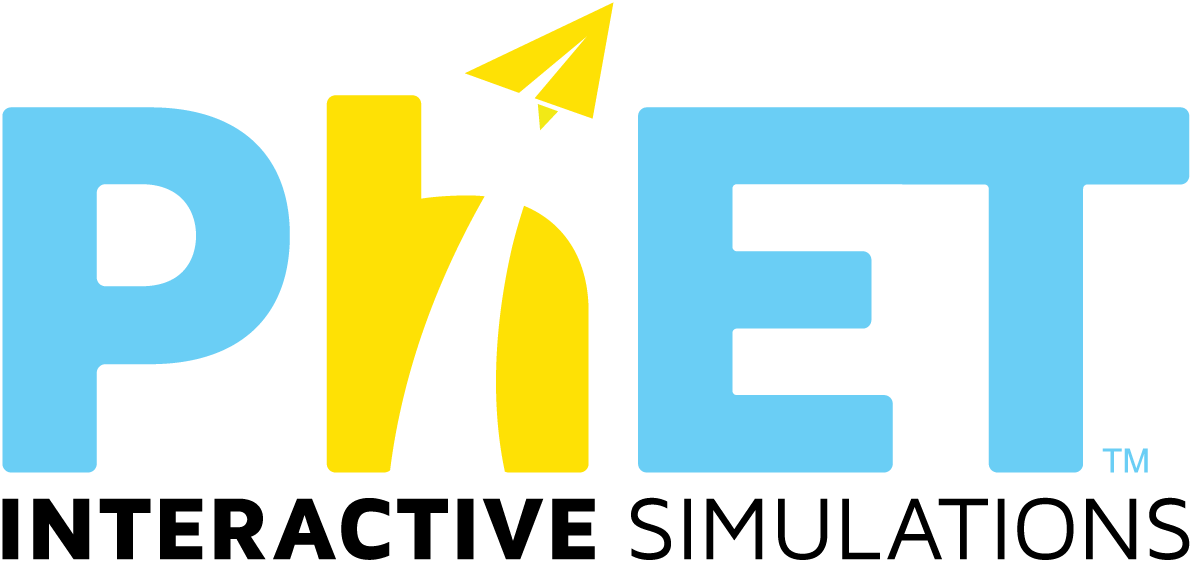
- Sign in / Register
- Administration
- Edit profile
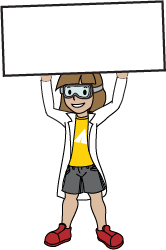
Exploring Light as a Wave
- Exploring-Light-as-a-wave-20210126.docx - 289 kB
Exploring Light as a Wave | |
A guided exploration starting with diffraction, moving to interference, then to slits to explore how light behaves like a wave. Includes steps where the student will have to determine how to measure wavelength using the tools in the simulation and calculate frequency. | |
Physics | |
High School, Middle School | |
Guided Activity, Homework, Lab, Remote Learning | |
30 minutes | |
No | |
English | |
, , , , , , , | |