| Instructor Resources | | |
| | | Course-wide Content | | |
|
 - Solar Energy Technologies Office
- Fellowships
- Contact SETO
- Funding Programs
- National Laboratory Research and Funding
- Solar Technical Assistance
- Prizes and Challenges
- Cross-Office Funding Programs
- Concentrating Solar-Thermal Power Basics
- Photovoltaic Technology Basics
- Soft Costs Basics
- Systems Integration Basics
- Concentrating Solar-Thermal Power
- Manufacturing and Competitiveness
- Photovoltaics
- Systems Integration
- Equitable Access to Solar Energy
- Solar Workforce Development
- Solar Energy Research Database
- Solar Energy for Consumers
- Solar Energy for Government Officials
- Solar Energy for Job Seekers
- Solar Energy for Professionals
- Success Stories
You’ve seen them on rooftops, in fields, along roadsides, and you’ll be seeing more of them: Solar photovoltaic (PV) installations are on the rise across the country—but how do they turn sunshine into energy? Simple answer: with semiconductors. Of course, there’s more to it. Understanding how solar cells work is the foundation for understanding the research and development projects funded by the U.S. Department of Energy’s Solar Energy Technologies Office (SETO) to advance PV technologies. PV has made rapid progress in the past 20 years, yielding better efficiency, improved durability, and lower costs. But before we explain how solar cells work, know that solar cells that are strung together make a module , and when modules are connected, they make a solar system , or installation . A typical residential rooftop solar system has about 30 modules. 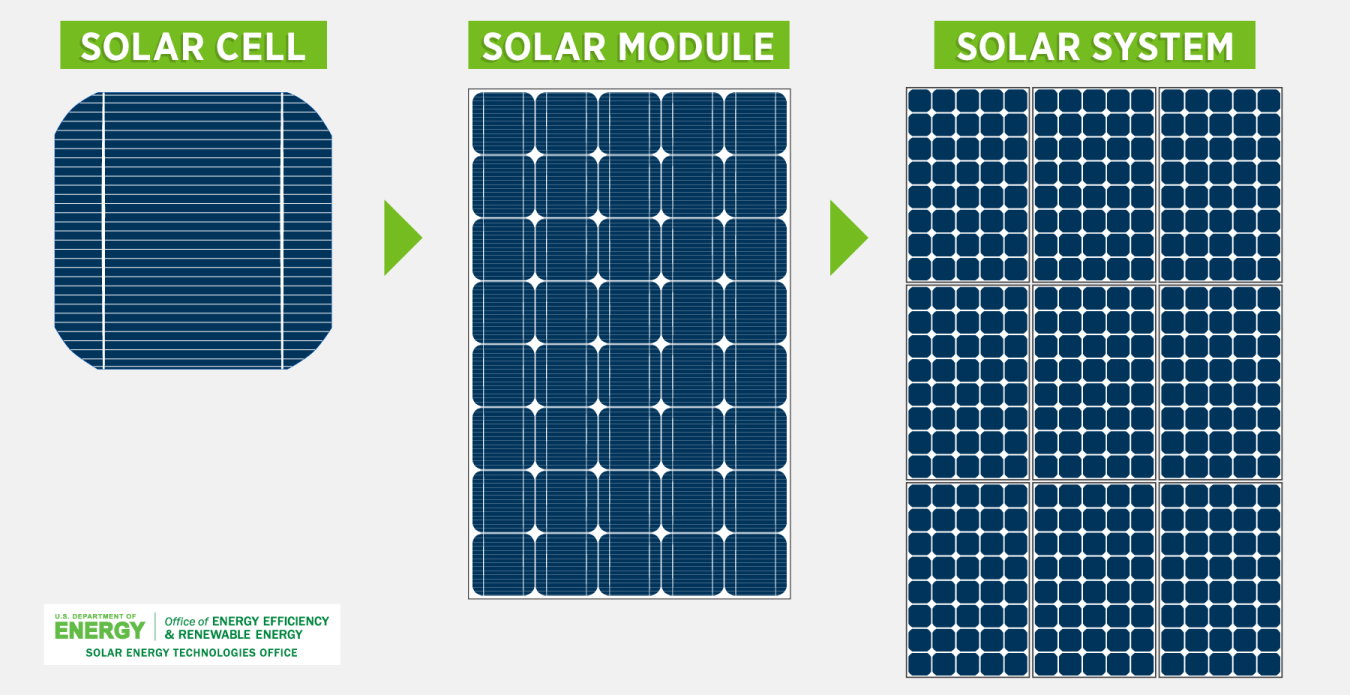 Now we can get down to business. How a Solar Cell WorksSolar cells contain a material that conducts electricity only when energy is provided—by sunlight, in this case. This material is called a semiconductor; the “semi” means its electrical conductivity is less than that of a metal but more than an insulator’s. When the semiconductor is exposed to sunlight, it absorbs the light, transferring the energy to negatively charged particles called electrons. The electrons flow through the semiconductor as electrical current, because other layers of the PV cell are designed to extract the current from the semiconductor. Then the current flows through metal contacts—the grid-like lines on a solar cell—before it travels to an inverter. The inverter converts the direct current (DC) to an alternating current (AC), which flows into the electric grid and, eventually, connects to the circuit that is your home’s electrical system. As long as sunlight continues to reach the module and the circuit is connected, electricity will continue to be generated. A module’s ability to convert sunlight into electricity depends on the semiconductor. In the lab, this ability is called photovoltaic conversion efficiency. Outside, environmental conditions like heat, dirt, and shade can reduce conversion efficiency, along with other factors . But researchers are coming up with solutions , such as backsheets that are placed on the panels to reduce their operating temperature, and new cell designs that capture more light. Capturing more light during the day increases energy yield , or the electricity output of a PV system over time. To boost energy yield, researchers and manufacturers are looking at bifacial solar cells, which are double-sided to capture light on both sides of a silicon solar module—they capture light reflected off the ground or roof where the panels are installed. The jury is still out on how bifacials will affect a system’s energy yield, but some SETO-funded projects are working to reduce this uncertainty by establishing baseline metrics to quantify and model bifacial efficiency gains . Silicon: The Market LeaderThe main semiconductor used in solar cells, not to mention most electronics, is silicon, an abundant element. In fact, it’s found in sand, so it’s inexpensive, but it needs to be refined in a chemical process before it can be turned into crystalline silicon and conduct electricity. Part 2 of this primer will cover other PV cell materials. To make a silicon solar cell, blocks of crystalline silicon are cut into very thin wafers. The wafer is processed on both sides to separate the electrical charges and form a diode, a device that allows current to flow in only one direction. The diode is sandwiched between metal contacts to let the electrical current easily flow out of the cell. About 95% of solar panels on the market today use either monocrystalline silicon or polycrystalline silicon as the semiconductor. Monocrystalline silicon wafers are made up of one crystal structure, and polycrystalline silicon is made up of lots of different crystals. Monocrystalline panels are more efficient because the electrons move more freely to generate electricity, but polycrystalline cells are less expensive to manufacture. The maximum theoretical efficiency level for a silicon solar cell is about 32% because of the portion of sunlight the silicon semiconductor is able to absorb above the bandgap —a property discussed in Part 2 of this primer. The best panels for commercial use have efficiencies around 18% to 22%, but researchers are studying how to improve efficiency and energy yield while keeping production costs low. Read more about solar PV research directions in Part 2 ! Thank you for visiting nature.com. You are using a browser version with limited support for CSS. To obtain the best experience, we recommend you use a more up to date browser (or turn off compatibility mode in Internet Explorer). In the meantime, to ensure continued support, we are displaying the site without styles and JavaScript. - View all journals
- Explore content
- About the journal
- Publish with us
- Sign up for alerts
- Published: 28 March 2019
Photovoltaic solar cell technologies: analysing the state of the art- Pabitra K. Nayak ORCID: orcid.org/0000-0002-7845-5318 1 ,
- Suhas Mahesh 1 ,
- Henry J. Snaith ORCID: orcid.org/0000-0001-8511-790X 1 &
- David Cahen ORCID: orcid.org/0000-0001-8118-5446 2
Nature Reviews Materials volume 4 , pages 269–285 ( 2019 ) Cite this article 22k Accesses 795 Citations 51 Altmetric Metrics details - Semiconductors
- Solar cells
- Solar energy and photovoltaic technology
The remarkable development in photovoltaic (PV) technologies over the past 5 years calls for a renewed assessment of their performance and potential for future progress. Here, we analyse the progress in cells and modules based on single-crystalline GaAs, Si, GaInP and InP, multicrystalline Si as well as thin films of polycrystalline CdTe and CuIn x Ga 1− x Se 2 . In addition, we analyse the PV developments of the more recently emerged lead halide perovskites together with notable improvements in sustainable chalcogenides, organic PVs and quantum dots technologies. In addition to power conversion efficiencies, we consider many of the factors that affect power output for each cell type and note improvements in control over the optoelectronic quality of PV-relevant materials and interfaces and the discovery of new material properties. By comparing PV cell parameters across technologies, we appraise how far each technology may progress in the near future. Although accurate or revolutionary developments cannot be predicted, cross-fertilization between technologies often occurs, making achievements in one cell type an indicator of evolutionary developments in others. This knowledge transfer is timely, as the development of metal halide perovskites is helping to unite previously disparate, technology-focused strands of PV research. This is a preview of subscription content, access via your institution Access optionsAccess Nature and 54 other Nature Portfolio journals Get Nature+, our best-value online-access subscription 24,99 € / 30 days cancel any time Subscribe to this journal Receive 12 digital issues and online access to articles 111,21 € per year only 9,27 € per issue Buy this article - Purchase on SpringerLink
- Instant access to full article PDF
Prices may be subject to local taxes which are calculated during checkout 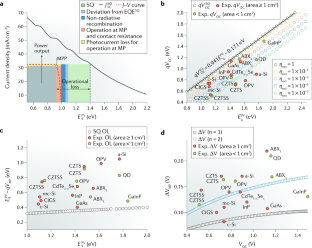 Similar content being viewed by others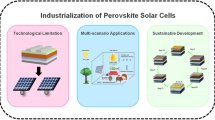 Achievements, challenges, and future prospects for industrialization of perovskite solar cells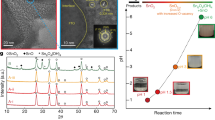 Efficient perovskite solar cells via improved carrier management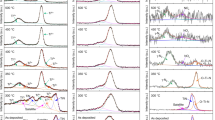 Centimetre-scale perovskite solar cells with fill factors of more than 86 per centShockley, W. & Queisser, H. J. Detailed balance limit of efficiency of p–n junction solar cells. J. Appl. Phys. 32 , 510–519 (1961). Article CAS Google Scholar Nayak, P. K., Bisquert, J. & Cahen, D. Assessing possibilities and limits for solar cells. Adv. Mater. 23 , 2870–2876 (2011). This study introduces operational loss as a parameter for the comparison and analysis of solar cell technologies . Nayak, P. K. & Cahen, D. Updated assessment of possibilities and limits for solar cells. Adv. Mater. 26 , 1622–1628 (2014). Rau, U., Blank, B., Müller, T. C. M. & Kirchartz, T. Efficiency potential of photovoltaic materials and devices unveiled by detailed-balance analysis. Phys. Rev. Appl. 7 , 044016 (2017). This study introduces the concept of determining the photovoltaic gap of a solar cell from the EQE of the cell . Article Google Scholar Wang, Y. et al. Optical gaps of organic solar cells as a reference for comparing voltage losses. Adv. Energy Mater. 8 , 1801352 (2018). Markvart, T. The thermodynamics of optical étendue. J. Opt. A 10 , 015008 (2008). Hirst, L. C. & Ekins-Daukes, N. J. Fundamental losses in solar cells. Prog. Photovolt. 19 , 286–293 (2011). This article provides analytical expressions for the fundamental losses in solar cells . Miller, O. D., Yablonovitch, E. & Kurtz, S. R. Strong internal and external luminescence as solar cells approach the Shockley–Queisser limit. IEEE J. Photovolt. 2 , 303–311 (2012). Rau, U. Reciprocity relation between photovoltaic quantum efficiency and electroluminescent emission of solar cells. Phys. Rev. B 76 , 085303 (2007). Green, M. A. et al. Solar cell efficiency tables (version 53). Prog. Photovolt. 27 , 3–12 (2019). This article provides solar cell parameters for the state-of-the-art cells . Schnitzer, I., Yablonovitch, E., Caneau, C. & Gmitter, T. J. Ultrahigh spontaneous emission quantum efficiency, 99.7% internally and 72% externally, from AlGaAs/GaAs/AlGaAs double heterostructures. Appl. Phys. Lett. 62 , 131–133 (1993). Green, M. A. Radiative efficiency of state-of-the-art photovoltaic cells. Prog. Photovolt. 20 , 472–476 (2012). Sheng, X. et al. Device architectures for enhanced photon recycling in thin-film multijunction solar cells. Adv. Energy Mater. 5 , 1400919 (2015). Geisz, J. F., Steiner, M. A., García, I., Kurtz, S. R. & Friedman, D. J. Enhanced external radiative efficiency for 20.8% efficient single-junction GaInP solar cells. Appl. Phys. Lett. 103 , 041118 (2013). Steiner, M. A. et al. CuPt ordering in high bandgap Ga x In 1- x P alloys on relaxed GaAsP step grades. J. Appl. Phys. 106 , 063525 (2009). Green, M. A. et al. Solar cell efficiency tables (version 49). Prog. Photovolt. 25 , 3–13 (2017). Wanlass, M. Systems and methods for advanced ultra-high-performance InP solar cells. US Patent US9590131B2 (2014). Green, M. A., Emery, K., Hishikawa, Y., Warta, W. & Dunlop, E. D. Solar cell efficiency tables (version 42). Prog. Photovolt. 21 , 827–837 (2013). Yoshikawa, K. et al. Silicon heterojunction solar cell with interdigitated back contacts for a photoconversion efficiency over 26%. Nat. Energy 2 , 17032 (2017). This study presents an efficient (PCE = 26.6%) c-Si solar cell with the IBC–SHJ architecture . Green, M. A. et al. Solar cell efficiency tables (version 52). Prog. Photovolt. 26 , 427–436 (2018). Taguchi, M. et al. 24.7% record efficiency HIT solar cell on thin silicon wafer. IEEE J. Photovolt. 4 , 96–99 (2014). Richter, A., Hermle, M. & Glunz, S. W. Reassessment of the limiting efficiency for crystalline silicon solar cells. IEEE J. Photovolt. 3 , 1184–1191 (2013). Trupke, T., Zhao, J., Wang, A., Corkish, R. & Green, M. A. Very efficient light emission from bulk crystalline silicon. Appl. Phys. Lett. 82 , 2996–44107 (2003). Yang, Y. M. et al. Development of high-performance multicrystalline silicon for photovoltaic industry. Prog. Photovolt. 23 , 340–351 (2015). Macdonald, D. & Geerligs, L. J. Recombination activity of interstitial iron and other transition metal point defects in p- and n-type crystalline silicon. Appl. Phys. Lett. 85 , 4061–4063 (2004). Benick, J. et al. High-efficiency n-type HP mc silicon solar cells. IEEE J. Photovolt. 7 , 1171–1175 (2017). Chirilă, A. et al. Potassium-induced surface modification of Cu(In,Ga)Se 2 thin films for high-efficiency solar cells. Nat. Mater. 12 , 1107–1111 (2013). Chantana, J., Kato, T., Sugimoto, H. & Minemoto, T. Thin-film Cu(In,Ga)(Se,S) 2 -based solar cell with (Cd,Zn)S buffer layer and Zn 1− x Mg x O window layer. Prog. Photovolt. 25 , 431–440 (2017). Kato, T., Wu, J.-L., Hirai, Y., Sugimoto, H. & Bermudez, V. Record efficiency for thin-film polycrystalline solar cells up to 22.9% achieved by Cs-treated Cu(In,Ga)(Se,S) 2 . IEEE J. Photovolt. 9 , 325–330 (2018). IEEE Electron Devices Society. IEEE Electron Devices Society Newsletter: highlights of the 2017 IEEE Photovoltaic Specialists Conference. IEEE https://eds.ieee.org/images/files/newsletters/newsletter_oct17.pdf (2017). Poplawsky, J. D. et al. Structural and compositional dependence of the CdTe x Se 1− x alloy layer photoactivity in CdTe-based solar cells. Nat. Commun. 7 , 12537 (2016). Paudel, N. R., Poplawsky, J. D., Moore, K. L. & Yan, Y. Current enhancement of CdTe-based solar cells. IEEE J. Photovolt. 5 , 1492–1496 (2015). Zhao, Y. et al. Monocrystalline CdTe solar cells with open-circuit voltage over 1 V and efficiency of 17%. Nat. Energy 1 , 16067 (2016). Gloeckler, M., Sankin, I. & Zhao, Z. CdTe solar cells at the threshold to 20% efficiency. IEEE J. Photovolt. 3 , 1389–1393 (2013). Lokanc, M., Eggert, R. & Redlinger, M. The availability of indium: the present, medium term, and long term. NREL https://www.nrel.gov/docs/fy16osti/62409.pdf (2015). Gokmen, T., Gunawan, O., Todorov, T. K. & Mitzi, D. B. Band tailing and efficiency limitation in kesterite solar cells. Appl. Phys. Lett. 103 , 103506 (2013). Ng, T. M. et al. Optoelectronic and spectroscopic characterization of vapour-transport grown Cu 2 ZnSnS 4 single crystals. J. Mater. Chem. A 5 , 1192–1200 (2017). Yan, C. et al. Beyond 11% efficient sulfide kesterite Cu 2 Zn x Cd 1− x SnS 4 solar cell: effects of cadmium alloying. ACS Energy Lett. 2 , 930–936 (2017). Kronik, L., Cahen, D. & Schock, H. W. Effects of sodium on polycrystalline Cu(In, Ga)Se 2 and its solar cell performance. Adv. Mater. 10 , 31–36 (1998). Nayak, P. K., Garcia-Belmonte, G., Kahn, A., Bisquert, J. & Cahen, D. Photovoltaic efficiency limits and material disorder. Energy Environ. Sci. 5 , 6022 (2012). Kim, S., Park, J. S. & Walsh, A. Identification of killer defects in kesterite thin-film solar cells. ACS Energy Lett. 3 , 496–500 (2018). Snaith, H. J. Present status and future prospects of perovskite photovoltaics. Nat. Mater. 17 , 372–376 (2018). This is a recent review on halide perovskite materials for optoelectronic applications . Stranks, S. D. et al. Electron-hole diffusion lengths exceeding 1 micrometer in an organometal trihalide perovskite absorber. Science 342 , 341–344 (2013). Edri, E. et al. Elucidating the charge carrier separation and working mechanism of CH 3 NH 3 PbI 3− x Cl x perovskite solar cells. Nat. Commun. 5 , 3461 (2014). Ceratti, D. R. et al. Self-healing inside APbBr 3 halide perovskite crystals. Adv. Mater. 30 , 1706273 (2018). Brandt, R. E., Stevanovic, V., Ginley, D. S. & Buonassisi, T. Identifying defect-tolerant semiconductors with high minority-carrier lifetimes: beyond hybrid lead halide perovskites. MRS Commun. 5 , 265–275 (2015). Zakutayev, A. et al. Defect tolerant semiconductors for solar energy conversion. J. Phys. Chem. Lett. 5 , 1117–1125 (2014). De Wolf, S. et al. Organometallic halide perovskites: sharp optical absorption edge and its relation to photovoltaic performance. J. Phys. Chem. Lett. 5 , 1035–1039 (2014). Sutter-Fella, C. M. et al. Band tailing and deep defect states in CH 3 NH 3 Pb(I 1− x Br x ) 3 perovskites as revealed by sub-bandgap photocurrent. ACS Energy Lett. 2 , 709–715 (2017). Braly, I. L. et al. Hybrid perovskite films approaching the radiative limit with over 90% photoluminescence quantum efficiency. Nat. Photonics 12 , 355–361 (2018). Tiedje, T. Band tail recombination limit to the output voltage of amorphous silicon solar cells. Appl. Phys. Lett. 40 , 627–629 (1982). This article demonstrates the effect of tail states on the efficiency of solar cells . Liu, M. et al. Hybrid organic–inorganic inks flatten the energy landscape in colloidal quantum dot solids. Nat. Mater. 16 , 258–263 (2017). Swarnkar, A. et al. Quantum dot-induced phase stabilization of α-CsPbI 3 perovskite for high-efficiency photovoltaics. Science 354 , 92–95 (2016). Sanehira, E. M. et al. Enhanced mobility CsPbI 3 quantum dot arrays for record-efficiency, high-voltage photovoltaic cells. Sci. Adv. 3 , eaao4204 (2017). Mori, S. et al. Organic photovoltaic module development with inverted device structure. Mater. Res. Soc. Symp. Proc. 1737 , 26–31 (2015). Yan, C. et al. Non-fullerene acceptors for organic solar cells. Nat. Rev. Mater. 3 , 18003 (2018). Marcus, R. A. Electron transfer reactions in chemistry. Theory and experiment. Rev. Mod. Phys. 65 , 599–610 (1993). Benduhn, J. et al. Intrinsic non-radiative voltage losses in fullerene-based organic solar cells. Nat. Energy 2 , 17053 (2017). Nayak, P. K. et al. The effect of structural order on solar cell parameters, as illustrated in a SiC-organic junction model. Energy Environ. Sci. 6 , 3272 (2013). Qian, D. et al. Design rules for minimizing voltage losses in high-efficiency organic solar cells. Nat. Mater. 17 , 703–709 (2018). Chen, X. K. & Brédas, J. L. Voltage losses in organic solar cells: understanding the contributions of intramolecular vibrations to nonradiative recombinations. Adv. Energy Mater. 8 , 1702227 (2018). Jean, J. et al. Radiative efficiency limit with band tailing exceeds 30% for quantum dot solar cells. ACS Energy Lett. 2 , 2616–2624 (2017). Venkateshvaran, D. et al. Approaching disorder-free transport in high-mobility conjugated polymers. Nature 515 , 384–388 (2014). Green, M. A. Accuracy of analytical expressions for solar cell fill factors. Solar Cells 7 , 337–340 (1982). Oxford PV. Oxford PV perovskite solar cell achieves 28% efficiency. Oxford PV https://www.oxfordpv.com/news/oxford-pv-perovskite-solar-cell-achieves-28-efficiency (2018). Haxel, G. B., Hedrick, J. B. & Orris, G. J. Rare earth elements: critical resources for high technology: US Geological Survey fact sheet 087–02. USGS https://pubs.usgs.gov/fs/2002/fs087-02/ (updated 17 May 2005). Chuangchote, S. et al. Review of environmental, health and safety of CdTe photovoltaic installations throughout their life-cycle. First Solar http://www.firstsolar.com/-/media/First-Solar/Sustainability-Documents/Sustainability-Peer-Reviews/Thai-EHS-Peer-Review_EN.ashx (2012). CHEOPS. First results regarding the environmental impact of perovskite/silicon tandem PV modules. CHEOPS https://www.cheops-project.eu/news-in-brief/first-results-regarding-the-environmental-impact-of-perovskitesilicon-tandem-pv-modules (2017). Meng, L. et al. Organic and solution-processed tandem solar cells with 17.3% efficiency. Science 361 , eaat2612 (2018). Ekins-Daukes, N. J. & Hirst, L. C. in 24th European Photovoltaic Solar Energy Conf . 457–461 (WIP-Munich, 2009). Green, M. A., Emery, K., Hishikawa, Y., Warta, W. & Dunlop, E. D. Solar cell efficiency tables (version 40). Prog. Photovolt. 20 , 606–614 (2012). Green, M. A., Emery, K., Hishikawa, Y., Warta, W. & Dunlop, E. D. Solar cell efficiency tables (version 47). Prog. Photovolt. 24 , 3–11 (2016). Adachi, D., Hernández, J. L. & Yamamoto, K. Impact of carrier recombination on fill factor for large area heterojunction crystalline silicon solar cell with 25.1% efficiency. Appl. Phys. Lett. 107 , 233506 (2015). Green, M. A. et al. Solar cell efficiency tables (version 50). Prog. Photovolt. 25 , 668–676 (2017). Kojima, A., Teshima, K., Shirai, Y. & Miyasaka, T. Organometal halide perovskites as visible-light sensitizers for photovoltaic cells. J. Am. Chem. Soc. 131 , 6050–6051 (2009). Kim, H.-S. et al. Lead iodide perovskite sensitized all-solid-state submicron thin film mesoscopic solar cell with efficiency exceeding 9%. Sci. Rep. 2 , 591 (2012). Lee, M. M., Teuscher, J., Miyasaka, T., Murakami, T. N. & Snaith, H. J. Efficient hybrid solar cells based on meso-superstructured organometal halide perovskites. Science 338 , 643–647 (2012). Gong, W. et al. Influence of energetic disorder on electroluminescence emission in polymer: fullerene solar cells. Phys. Rev. B 86 , 024201 (2012). Liu, J. et al. Fast charge separation in a non-fullerene organic solar cell with a small driving force. Nat. Energy 1 , 16089 (2016). Green, M. A., Emery, K., Hishikawa, Y., Warta, W. & Dunlop, E. D. Solar cell efficiency tables (version 44). Prog. Photovolt. 22 , 701–710 (2014). Green, M. A. et al. Solar cell efficiency tables (version 51). Prog. Photovolt. 26 , 3–12 (2018). Green, M. A., Emery, K., Hishikawa, Y., Warta, W. & Dunlop, E. D. Solar cell efficiency tables (Version 45). Prog. Photovolt. 23 , 1–9 (2015). Download references AcknowledgementsThe authors acknowledge the support from the UK Engineering and Physical Sciences Research Council (grant nos EP/P032591/1 and EP/M015254/2) and thank B. Wenger, T. Markvardt, T. Kirchartz, T. Buonassisi and A. Bakulin for critical comments and data and D. Friedman for providing a GaAs cell. D.C. thanks the Weizmann Institute of Science, where he held the Rowland and Sylvia Schaefer Chair in Energy Research, for partial support. Author informationAuthors and affiliations. Clarendon Laboratory, Department of Physics, University of Oxford, Oxford, UK Pabitra K. Nayak, Suhas Mahesh & Henry J. Snaith Department of Materials and Interfaces, Weizmann Institute of Science, Rehovot, Israel David Cahen You can also search for this author in PubMed Google Scholar ContributionsAll authors contributed to the discussion of content. P.K.N. researched most of the data, carried out the analysis and wrote the article. D.C. and S.M. contributed to the researching of data and analysis. D.C., H.J.S. and P.K.N. edited the manuscript before submission. Corresponding authorCorrespondence to Pabitra K. Nayak . Ethics declarationsCompeting interests. H.J.S. is the co-founder and CSO of Oxford PV Ltd, a company that is commercializing perovskite photovoltaic technologies. P.K.N., S.M. and D.C. declare no competing interests. Additional informationPublisher’s note. Springer Nature remains neutral with regard to jurisdictional claims in published maps and institutional affiliations. Related linksReference Solar Spectral Irradiance: Air Mass 1.5 : https://rredc.nrel.gov/solar//spectra/am1.5/#about Supplementary informationSupplementary information, rights and permissions. Reprints and permissions About this articleCite this article. Nayak, P.K., Mahesh, S., Snaith, H.J. et al. Photovoltaic solar cell technologies: analysing the state of the art. Nat Rev Mater 4 , 269–285 (2019). https://doi.org/10.1038/s41578-019-0097-0 Download citation Published : 28 March 2019 Issue Date : April 2019 DOI : https://doi.org/10.1038/s41578-019-0097-0 Share this articleAnyone you share the following link with will be able to read this content: Sorry, a shareable link is not currently available for this article. Provided by the Springer Nature SharedIt content-sharing initiative This article is cited byTerahertz photon to dc current conversion via magnetic excitations of multiferroics. - Makiko Ogino
- Yoshihiro Okamura
- Youtarou Takahashi
Nature Communications (2024) A complementary step to halide perovskite electronicsNature Electronics (2024) Integrated triboelectric nanogenerator and radiative cooler for all-weather transparent glass surfacesRegulating phase homogeneity by self-assembled molecules for enhanced efficiency and stability of inverted perovskite solar cellsNature Photonics (2024) High carrier mobility along the [111] orientation in Cu2O photoelectrodes- Linfeng Pan
- Samuel D. Stranks
Nature (2024) Quick links- Explore articles by subject
- Guide to authors
- Editorial policies
Sign up for the Nature Briefing newsletter — what matters in science, free to your inbox daily.  Solar Energy And Photovoltaic CellWe have learned about the role of renewable energy in the sustainable development of the country. Renewable energy is more sustainable than fossil fuel sources. Sun is the source of renewable energy. The radiating light and heat from the sun are harnessed and converted into other forms of energy. In this article let us learn about solar power, solar energy, and photovoltaic cells in detail. Solar Power:Solar power is an indefinitely renewable source of energy as the sun has been radiating an estimated 5000 trillion kWh of energy for billions of years and will continue to do so for the next 4 billion years. Solar energy is a form of energy which is used in power cookers, water heaters etc. The primary disadvantage of solar power is that it cannot be produced in the absence of sunlight. This limitation is overcome by the use of solar cells that convert solar energy into electrical energy. In this section, we will learn about the photovoltaic cell, its advantages, and disadvantages. 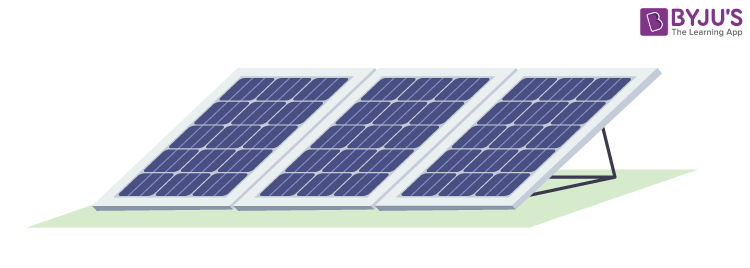 Solar Energy:It is defined as the radiating light and heat from the sun that is harnessed using devices like heaters, solar cookers, and photovoltaic cells to convert it to other forms of energy such as electrical energy and heat. 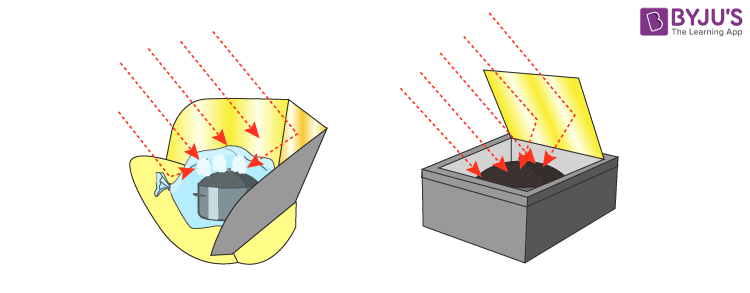 Photovoltaic Cell:- Photovoltaic cells consist of two or more layers of semiconductors with one layer containing positive charge and the other negative charge lined adjacent to each other.
- Sunlight, consisting of small packets of energy termed as photons, strikes the cell, where it is either reflected, transmitted or absorbed.
- When the photons are absorbed by the negative layer of the photovoltaic cell, the energy of the photon gets transferred to an electron in an atom of the cell.
- With the increase in energy, the electron escapes the outer shell of the atom. The freed electron naturally migrates to the positive layer creating a potential difference between the positive and the negative layer. When the two layers are connected to an external circuit, the electron flows through the circuit, creating a current.
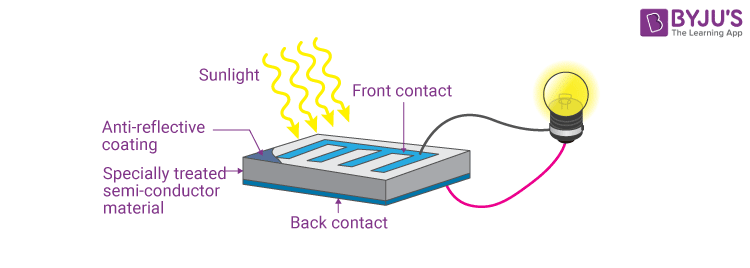 Advantages of Photovoltaic Cells:- Environmental Sustainability: Photovoltaic cells generate clean and green energy as no harmful gases such as CO x , NO x etc are emitted. Also, they produce no noise pollution which makes them ideal for application in residential areas.
- Economically Viable: The operation and maintenance costs of cells are very low. The cost of solar panels incurred is only the initial cost i.e., purchase and installation.
- Accessible: Solar panels are easy to set up and can be made accessible in remote locations or sparsely inhabited areas at a lesser cost as compared to conventional transmission lines. They are easy to install without any interference with the residential lifestyle.
- Renewable: Energy is free and abundant in nature.
- Cost: Solar panels have no mechanically moving parts except in some highly advanced sunlight tracking mechanical bases. Consequently, the solar panel price for maintenance and repair is negligible.
Disadvantages of Photovoltaic Cells:- The efficiency of solar panels is low compared to other renewable sources of energy.
- Energy from the sun is intermittent and unpredictable and can only be harnessed in the presence of sunlight. Also, the power generated gets reduced during cloudy weather.
- Long-range transmission of solar energy is inefficient and difficult to carry. The current produced is DC in nature and the conversion of DC current to AC current involves the use of additional equipment such as inverters.
- Photovoltaic panels are fragile and can be damaged relatively easily. Additional insurance costs are required to ensure a safeguard of the investments.
Frequently Asked Questions – FAQsHow do solar cells work, what is the principle of solar cells. Silicon crystals are laminated into p-type and n-type layers, stacked on top of each other. Light striking the crystals induces the “photovoltaic effect,” which generates electricity. State true or false: Solar energy is a renewable form of energy.What does the solar cell include, can solar power from photovoltaic cells be harnessed in the absence of sunlight. No, it can be only harnessed in the presence of sunlight. Hope you have learned in detail about Photovoltaic Cells along with advantages and disadvantages. Stay tuned with BYJU’S for more such interesting articles. 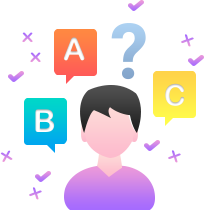 Put your understanding of this concept to test by answering a few MCQs. Click ‘Start Quiz’ to begin! Select the correct answer and click on the “Finish” button Check your score and answers at the end of the quiz Visit BYJU’S for all Physics related queries and study materials Your result is as below Request OTP on Voice Call Leave a Comment Cancel replyYour Mobile number and Email id will not be published. Required fields are marked * Post My Comment  Register with BYJU'S & Download Free PDFsRegister with byju's & watch live videos. InformationInitiativesYou are accessing a machine-readable page. In order to be human-readable, please install an RSS reader. All articles published by MDPI are made immediately available worldwide under an open access license. No special permission is required to reuse all or part of the article published by MDPI, including figures and tables. For articles published under an open access Creative Common CC BY license, any part of the article may be reused without permission provided that the original article is clearly cited. For more information, please refer to https://www.mdpi.com/openaccess . Feature papers represent the most advanced research with significant potential for high impact in the field. A Feature Paper should be a substantial original Article that involves several techniques or approaches, provides an outlook for future research directions and describes possible research applications. Feature papers are submitted upon individual invitation or recommendation by the scientific editors and must receive positive feedback from the reviewers. Editor’s Choice articles are based on recommendations by the scientific editors of MDPI journals from around the world. Editors select a small number of articles recently published in the journal that they believe will be particularly interesting to readers, or important in the respective research area. The aim is to provide a snapshot of some of the most exciting work published in the various research areas of the journal. Original Submission Date Received: . - Active Journals
- Find a Journal
- Journal Proposal
- Proceedings Series
- For Authors
- For Reviewers
- For Editors
- For Librarians
- For Publishers
- For Societies
- For Conference Organizers
- Open Access Policy
- Institutional Open Access Program
- Special Issues Guidelines
- Editorial Process
- Research and Publication Ethics
- Article Processing Charges
- Testimonials
- Preprints.org
- SciProfiles
- Encyclopedia
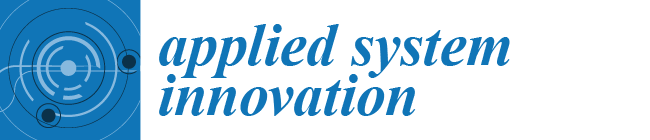 Article Menu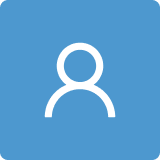 - Subscribe SciFeed
- Recommended Articles
- Google Scholar
- on Google Scholar
- Table of Contents
Find support for a specific problem in the support section of our website. Please let us know what you think of our products and services. Visit our dedicated information section to learn more about MDPI. JSmol ViewerPhotovoltaic solar cells: a review. 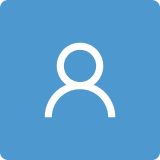 1. Introduction2. solar cells, 2.1. the working principle of pv cells. - Absorption of photons in a p-n junction electronic semiconductor to generate the charge carriers (electron-hole pairs). The absorption of a photon with energy (E = hυ) higher than the gap energy ‘E g ’ of the doped semiconductor material means that its energy is used to excite an electron from the valence band ‘Eυ’ to the conduction band ‘E c ’ leaving a void (hole) at the valance level. Additional kinetic energy is given to the electron or hole by the excess photon energy (hυ–hυ 0 ). ‘hυ 0 ′ is the minimum energy or work function of the semiconductor required to generate an electron-hole pair. The work function here represents the energy gap. The excess energy is dissipated as heat in the semiconductor [ 21 , 22 ].
- Consequent separation of the light-generated charge carriers. In an external solar circuit, the holes can flow away from the junction through the p-region, and electrons can flow out across the n-region and pass through the circuit before they recombine with the holes.
- Finally, the separated electrons can be used to drive an electric circuit. After the electrons passed through the circuit, they will recombine with the holes.
2.2. Solar Cell Panels2.3. components of solar power system, 2.4. p-n junction solar cell, 2.4.1. formation of the depletion region, 2.4.2. p-n junction solar cell under applied voltage, 2.4.3. pv cell under illumination. - The net flow of the electrons and holes in a p-n junction semiconductor under equilibrium conditions will generate two currents: ‘ I diff ’ and ‘ I drift ’. These currents balance and cancel each other at the equilibrium state.
- If an external source is deployed to the p-n junction, the generated current is the diode current ‘ I d ’.
- Under illumination, the p-n junction will present another current called light or photocurrent ‘ I ph ’.
2.5. I-V and P-V Characteristics- Short-circuit current density ‘ Isc ’ occurs at (R = 0 and V = 0)
- Open-circuit voltage ‘ Voc ’ (no-load, I = 0 and R = ∞)
- Fill factor ‘ FF ’ that represents the ratio of ‘ Pmax ’ to the electrical output of ‘ Voc ’ and ‘ Isc ’
2.6. Design Considerations2.7. materials employed in pv cells, 2.7.1. iii-v pv gallium arsenide, 2.7.2. future trends, 2.8. challenges in solar cells, 3. simulation of solar cells and modules, 3.1. simulation of solar cells by matlab/simulink, 3.2. simulation of solar cells by comsol/multiphysics. - Creating a user-defined, spatially dependent variable for the generation rate, using an integral expression involving the solar radiation ‘ F ( λ )’, which is used to find the rate of photon generation ‘ ϕ ( λ )’.
Share and CiteAl-Ezzi, A.S.; Ansari, M.N.M. Photovoltaic Solar Cells: A Review. Appl. Syst. Innov. 2022 , 5 , 67. https://doi.org/10.3390/asi5040067 Al-Ezzi AS, Ansari MNM. Photovoltaic Solar Cells: A Review. Applied System Innovation . 2022; 5(4):67. https://doi.org/10.3390/asi5040067 Al-Ezzi, Athil S., and Mohamed Nainar M. Ansari. 2022. "Photovoltaic Solar Cells: A Review" Applied System Innovation 5, no. 4: 67. https://doi.org/10.3390/asi5040067 Article MetricsArticle access statistics, further information, mdpi initiatives, follow mdpi. 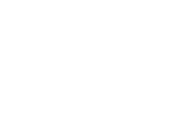 Subscribe to receive issue release notifications and newsletters from MDPI journals - Review PVcase Products
- Help Center
- Get in Touch
- Privacy Policy
- Cookie Policy
- Whistleblower Policy
- Exclusive Features
- Schedule Demo
- Customer Stories
- Content Library
- Get Free Trial
The biggest problems with solar power today, and how to solve themOver the past decade, the solar installation industry has experienced an average annual growth rate of 24% . A 2021 study by the National Renewable Energy Laboratory (NREL) projected that 40% of all power generation in the U.S. could come from solar by 2035. Solar’s current trends and forecasts look promising, with photovoltaic (PV) installations playing a major role in solving energy problems like carbon pollution and energy dependence. However, challenges related to solar energy threaten to slow growth and make solar less accessible to homeowners and businesses. These issues include problems connecting solar to electrical grids, equipment shortages, supply chain delays, a lack of land for commercial solar arrays, and a lack of qualified contractors and laborers to meet installation demands. Industry stakeholders, governments, manufacturers, and scientists are seeking ways to address these roadblocks and push the development of solar power forward. Here is a closer look at the issues affecting the PV sector and current efforts to solve them. Technological limitations in photovoltaic efficiencyThe U.S. Department of Energy defines solar conversion efficiency as “the percentage of the solar energy shining on a PV device that is converted into usable electricity.” The agency points out that most of the sunlight hitting PV cells gets lost during the conversion process. Light either gets reflected or turned into heat instead of getting converted into electricity. Recombination is another factor limiting PV efficiency. It happens when charge-carrying electrons encounter defects in the PV material or merge with charge carriers known as “holes,” which do not have electrons. During recombination, the energy turns into light photons or heat instead of producing energy. Currently, most panels have efficiencies of 17-20% . 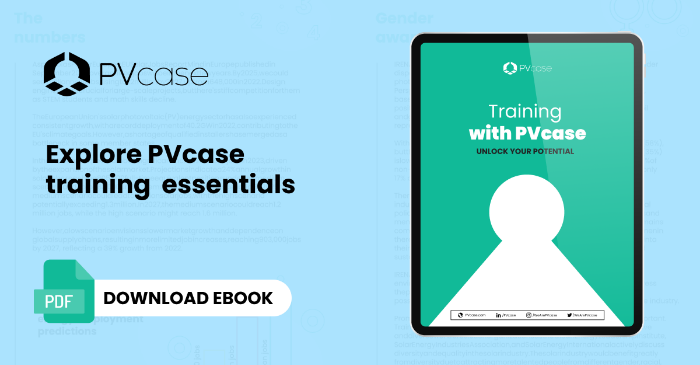 Solutions to improve PV efficiencyResearchers have developed new technologies, such as multi-junction PV cells , which increase efficiency to more than 45%. Manufacturing costs, material availability, and other factors need to be addressed before this technology becomes accessible for commercial use. Another solution is bifacial solar panels , which have cells on their underside to catch light reflected off the ground, roof, or other reflective surfaces. If combined with sun-tracking panels that adjust to maximize solar contact throughout the day, bifacial panels are 30-40% more efficient than their one-sided counterparts. Solar intermittency and storage challengesSolar intermittency is the most obvious issue related to PV panel efficiency. The sun is not visible for 24 hours per day except for a short time each year at extreme latitudes. Solar power users need other power sources to use after sunset, and utilities cannot rely on solar alone to provide electricity for their customers. One solution is to capture extra energy during the daytime and store it. However, storage issues are common. Batteries add to the cost of solar installation. Costs for batteries to cover home energy are $8,500 to $10,000 , not including installation and maintenance. These systems may not be enough to cover high energy usage periods, such as heating or cooling the home during extreme temperatures. Solutions to improve solar reliabilityOne of the most common solutions for residential and small business customers is net metering . Users keep their utility connections, and their system feeds unused energy back into the grid. The utility company offers credits that the customer can use to cover the cost of electricity from the grid after dark. Battery technologies are improving, and smart energy management software can help solar users maximize efficiency based on electricity usage patterns. Advances such as more efficient lithium-ion batteries and safer, faster-charging solid-state batteries are on the horizon. These could make storage more efficient and cost-effective and make it possible for more solar users to disconnect from the grid. Geographic variations in solar intensityIn general, the closer to the equator a location is, the more solar radiation it receives and the more energy PV cells can produce. However, pollution, cloud cover, foliage, elevation, and other factors also play a role in how much solar energy hits PV panels. In areas with low levels of solar radiation, such as locations in higher latitudes, solar panels may not produce as much energy, making them a less cost-effective option. The same could hold true for areas with lots of cloud cover and rainfall. 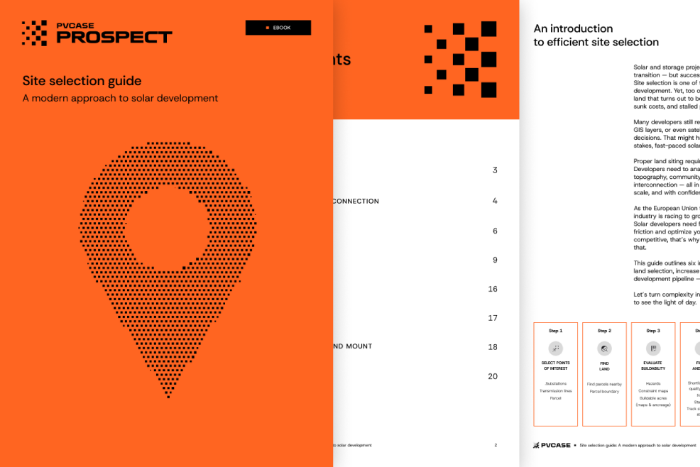 Solutions for solar intensity limitationsSolar optimization is one area that has already enjoyed significant advancements. For instance, solar tracking technology allows panels to adjust daily and seasonal changes in the sun’s location. Dual-axis panels rely on software to calculate the ideal angle and tilt based on available sunlight at a given time. Common in utility-scale ground-mounted systems, these systems ensure panels are always in the position to maximize solar radiation exposure. AI-powered software can also perform an advanced site analysis and use mathematical models to help optimize the placement, location, and angle of panels in a solar array. With these tools, you can design ground-mounted and rooftop systems and calculate yield to ensure maximum exposure to solar energy.  Cost competitiveness with other energy sourcesSolar energy itself is cost-effective. Systems produce free energy with limited maintenance for their lifespan, which averages 30 to 35 years. However, many people have to decide if solar is worth the investment. The reason for this indecision is the upfront cost of solar installation and the knowledge that they may still have to pay for supplemental power at times. EnergySage puts the cost of an average home solar PV system at $30,000, with the price dropping to about $21,000 if you take advantage of government incentives. This large initial investment may put solar out of reach for some customers and have others worrying about the return on their investment. Solutions for cost-effectivenessFederal and state incentives lower the overall cost of solar PV installation for both homeowners and businesses. However, other developments could help decrease costs. NREL documented a trend of significant price decline for solar installations during the 2010s, with most of the cost decrease due to lower panel and equipment costs. In 2021, the Department of Energy announced an effort to lower prices even further , targeting 60% cheaper installation costs by 2030. The DoE points to advances in promising technologies and low-cost materials, such as perovskite, as major cost-lowering factors. Also, an increase in domestic panel production will help avoid tariffs and geopolitical issues. Land use requirementsSolar panels require a large area for energy production. Utility-scale solar farms use at least 10 times as much land as coal and natural gas plants, including the land to extract and transport the fossil fuels, to produce a comparable amount of power. This has raised concerns in sectors like agriculture, with farmers expressing worries that the best farmland could be earmarked for solar developments. Finally, the DoE has also acknowledged concerns about how large arrays could affect wildlife, highlighting worries about water quality, bird collisions, and disruption to ecosystems. Solutions for land use concernsDesign and optimization are key elements of the effort to limit the size of large solar plants. Utility providers can use specialized software to measure the potential yield of solar arrays. These tools will allow energy companies to minimize area while maximizing output. Other advances focus on the novel field of agrivoltaics , which involves integrating solar into farmland without disrupting crop production. Solutions, like raised mounting systems and selection of shade-growing crops, are aspects of this effort, with some farmers finding advantages in the temperature regulation offered by solar panels to increase farm yields. 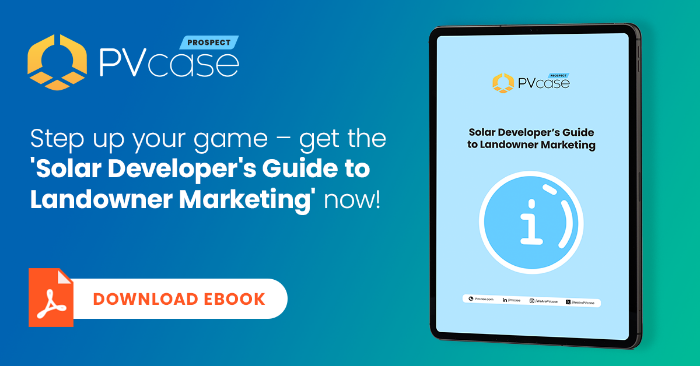 Solar panel life cycle and environmental impactSolar panels degrade over time, with the lifespan depending on their build quality, maintenance, and local conditions. Most panels retain 80% of their electricity production capacity after 30 years. However, after that, they need to be removed and replaced. Environmental advocates express concerns about the inability to recycle panels and the potentially hazardous materials that some contain. The environmental impact goes beyond waste. While the panels themselves produce energy without carbon emissions, other processes in the life cycle of a panel may not be as clean . Mining of necessary minerals and manufacturing panels may produce pollution, adding to the overall carbon footprint of solar energy. The same applies to transporting panels to installation locations. These factors increase the impact of panels on the environment, perhaps negating some of the benefits they provide. Solutions for reducing environmental impactRecent research has focused on solar panel recycling, with some studies producing promising results for the reuse of silicon from old solar panels. These developments could eventually lead to the reuse of materials, limiting waste. Meanwhile, NREL highlights research efforts that could increase the lifespan of solar panels to 50 years or more. This effort requires experimenting with different cell and module technologies and working on panel packaging. With the impressive growth of solar energy in recent years, the future looks promising . Researchers and companies are actively trying to address many of the problems slowing the solar PV industry, and governments continue to offer incentives and create policies that focus on making clean energy more accessible to everyone.  You might also be interested in: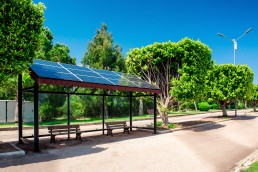 September 20, 2024 7 most expected innovations in shared mobilityShared mobility is a transportation solution that benefits individual travelers and communities. While many such solutions already exist, there are many areas where this type of…  September 19, 2024 The future of advanced materialsDive into the article to explore the latest advancements in advanced materials, their impact on industries, and the opportunities for businesses to stay ahead of the competition. 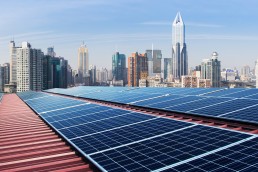 September 18, 2024 Are smart cities feasible?Smart cities offer many benefits compared to traditional urban infrastructure, but there are questions about their feasibility. Further investments in innovative technologies and… 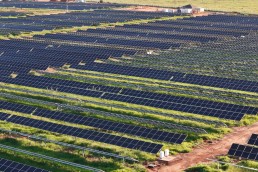 September 17, 2024 Challenges and solutions to financial services in rural areasMany people in rural areas have difficulty accessing financial services, a problem exacerbated by energy insecurity. Solar power can help protect rural communities from energy… 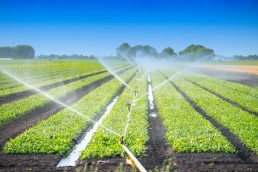 September 16, 2024 How to make climate-resilient crops even more sustainableLearn about the latest innovations and practices that can further improve the sustainability of climate-resilient crops, benefiting both farmers and the environment. 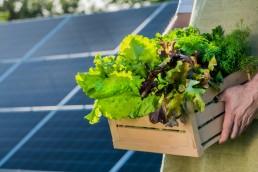 September 13, 2024 Emerging innovations in post-harvest technologyLearn about the latest innovations in post-harvest technology and how they are revolutionizing the agriculture industry. 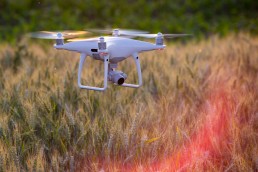 September 12, 2024 How precision agriculture can benefit both businesses and communitiesDiscover the advantages of precision agriculture for both businesses and communities, including increased efficiency and sustainability. Continue reading to find out. 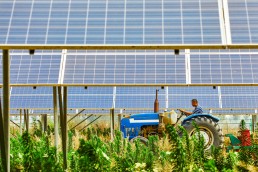 September 11, 2024 The expansion of agricultural tourism and its potential effectsDiscover how expanding agricultural tourism can benefit businesses, education, and local economies. Read on to learn more. 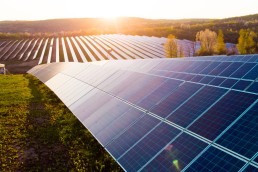 September 10, 2024 Why do GIS tools like ArcGIS/QGIS hold back your solar projects?Traditional platforms like ArcGIS and QGIS, while robust, were designed for GIS specialists in a different era. Find a solution that's more advanced to today's era, helping… 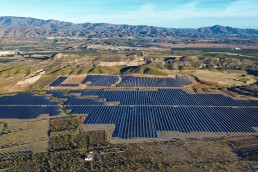 September 4, 2024 How did PVcase and GVC Ingegneria tackled daunting Italian terrain?GVC Ingegneria overcame technical challenges in developing PV project across the mountainous Italian terrain. Read the article to learn how did the company reach the maximum… 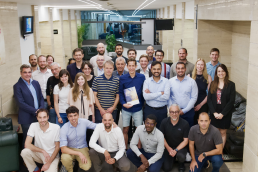 August 27, 2024 PVcase drives green innovation in EU’s landmark TRUST-PV projectThe multi-million TRUST-PV research project boosts the efficiency of distributed and utility-scale PV power plants, with PVcase innovating 3D digital twin-based modeling of solar… 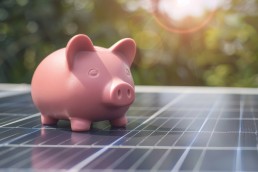 August 22, 2024 CapEx: how can developers reduce it?Read the article to learn effective strategies for developers to reduce CapEx, eliminating risks of budget overruns, project delays, and low profitability. 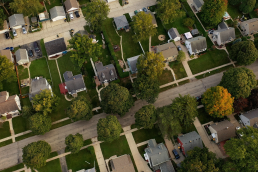 August 21, 2024 Understanding energy communities: exploring location-based categories under the IRAWhat is an energy community? There are three location-based categories under the IRA, each with qualifying criteria. Read on to learn more. 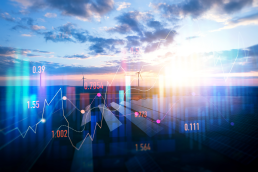 Selecting ideal parcels for renewable development. What to consider while using PVcase Prospect’s integrated data?Dive into the essential elements of property selection for renewable energy project with PVcase Prospect: from understanding renewable resource potential to assessing land… 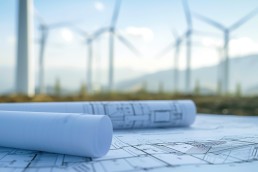 August 20, 2024 Grid capacity — the silent solar project killerLearn how grid capacity can silently hinder solar projects and find out more about PVcase Prospect's Capacity add-on that offers easy solutions to overcome these challenges.  Provide details on what you need help with along with a budget and time limit. Questions are posted anonymously and can be made 100% private.  Studypool matches you to the best tutor to help you with your question. Our tutors are highly qualified and vetted.  Your matched tutor provides personalized help according to your question details. Payment is made only after you have completed your 1-on-1 session and are satisfied with your session.  - Homework Q&A
- Become a Tutor
 All Subjects Mathematics Programming Health & Medical Engineering Computer Science Foreign Languages  Access over 35 million academic & study documentsPhotovoltaic cells case study.  Sign up to view the full document!  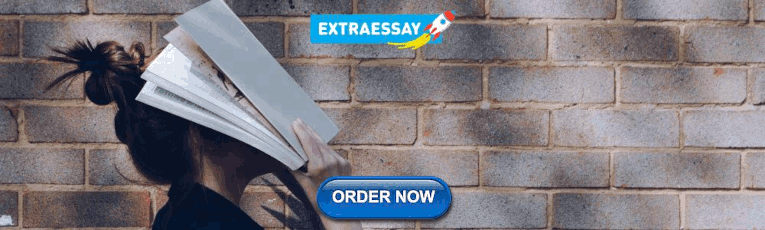 24/7 Study HelpStuck on a study question? Our verified tutors can answer all questions, from basic math to advanced rocket science !  Similar Documents working on a study question?  Studypool is powered by Microtutoring TM Copyright © 2024. Studypool Inc. Studypool is not sponsored or endorsed by any college or university. Ongoing Conversations Access over 35 million study documents through the notebank  Get on-demand Q&A study help from verified tutors  Read 1000s of rich book guides covering popular titles  Sign up with Google  Sign up with Facebook Already have an account? Login Login with Google Login with Facebook Don't have an account? Sign Up 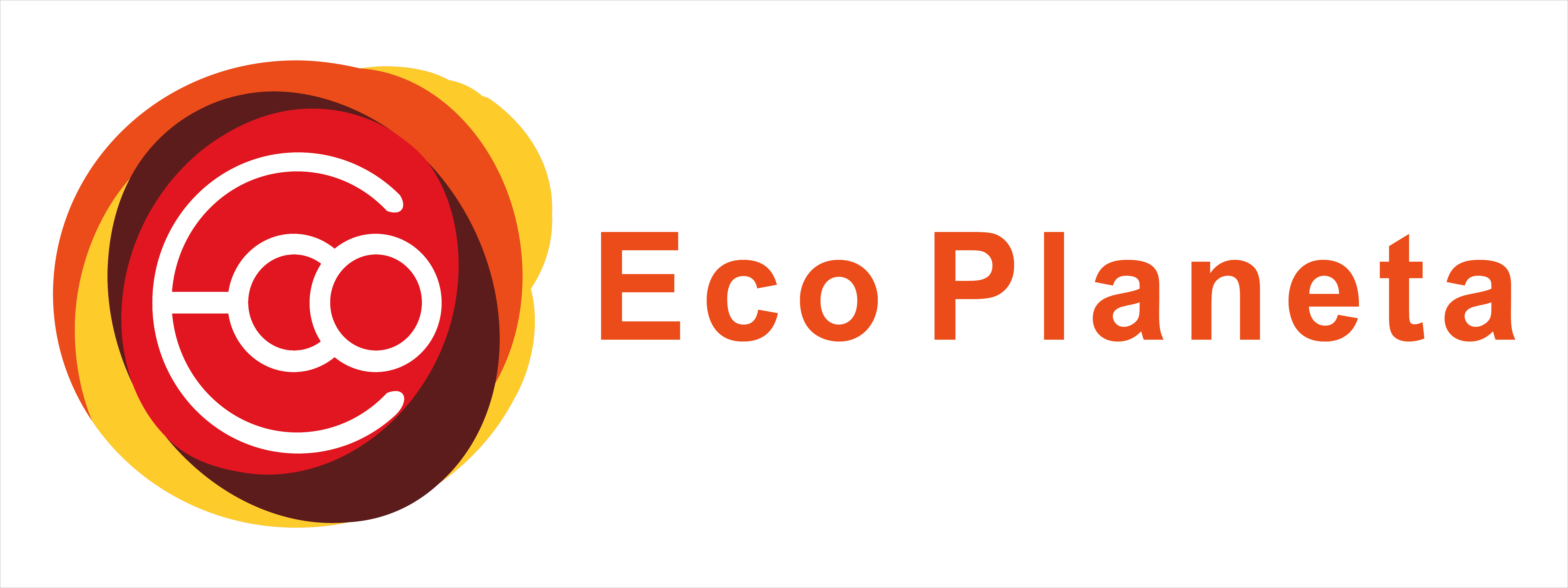 100 Best Solar Energy Case Studies of 2019The adoption of solar energy in the world is growing at a rapid pace in the world. More and more consumers, businesses and governmental organizations are considering solar energy. But it can be sometimes difficult to convince your family, friends, boss or colleagues to adopt solar energy? To make it easier to convince people to adopt solar power we selected the best and most complete 100 solar energy case studies. The case studies included in this list contain key information about the return on investment and annual savings of solar energy systems built all over the world and different sizes. The list is divided in three categories: Residential Solar EnergyCommercial solar energy, public sector solar energy, 1. home lavallee family. Country: Cumberland, Rhode Island, United States Installer: Renewable Energy Service of New England Inc. Solar PV: Suniva Inverter: Enphase Size: 9.5 kW Return on Investment: 34.9% Annual Savings: $3845 RES installed 33 solar modules for the Lavallee Family. The projected return of investment is 6 years. Read case study 2. Home Middle FranconiaCountry: Bavaria, Germany Inverters: SMA Size: 5 kWp Cost reduction: €875 per year One family of five installed a solar energy system with batteries. The whole system included a SMA pv inverter, a SMA battery inverter and a SMA sunny home manager for system monitoring and energy management. 3. Home Götz FamilyCountry: Wetzlar-Hermannstein, Germany Installer: Gecko Logic Solar PV: Yingli Inverters: SMA Size: 8.5 kWp Cost Reduction: €3936 per year A colleague convinced the family to invest in solar energy. The solar modules exceed the predicted energy yield. This system was installed by Gecko Logic. 4. Home Tan FamilyCountry: Jalan Kelawar, Tanglin, Singapore Installer: ReZeca Renewables Solar PV: Yingli Solar Size: 18.6 kWp Estimated Annual Savings: SGD$6000 The Tan Family wanted to reduce their footprint and their energy bills. In total 62 solar panels were installed. 5. Home Pappalardo FamilyCountry: Viagrande, Italy Installer: Etnergia Solar PV: Yingli Inverters: SMA Size: 8.58 kW Cost Reduction: €5533 per year After seeing solar pv installation in other countries the family decided to switch to solar energy. The company Etnergia installed 39 solar panels on roof with south-east orientation. The system is performing better than expected. 6. Absolute CoatingsCountry: New Rochelle, New York, United States Installer: Sunrise Solar Solutions Inverter: Enphase Size: 82 kW Savings over system life: $442 866 Sunrise Solar Solutions designed and installed 313 solar modules for Absolute Coatings on a new roof. The mounting system is ballast only. This project is part of 200 kW solar energy system that will completed in a next phase. 7. Rehme SteelCountry: Spicewood, United States Installer: Freedom Solar Power Solar PV: Sunpower Size: 81.6 kW Estimated savings over 25 years: $338 883 Rehme Steel wanted to reduce their operating cost and their carbon emissions. 8. Birkhof Horse Stables and Riding SchoolCountry: Waldsoms, Germany Installer: Gecko Logic Solar PV: Yingli Inverters: SMA Size: 34.68 kWp Cost Reduction: €12 954 Birkhof choose for solar energy, because of environmental and cost reduction reasons. Gecko Logic installed the system in 2008. 9. Ryan and Ryan InsuranceCountry: Kingston, New York, United States Installer: Sunrise Solar Solutions Solar PV: Conergy Inverter: Enphase Size: 16.3 kW Savings over lifetime system: $69 654 Years to breakeven: 5.9 The roof of Ryan and Ryan Insurance was big enough to place enough solar panels to cover their whole energy consumption. The solar panels are mounted with a fully ballasted racking system. 10. Powerplant PoggiorsiniCountry: Poggiorsini (Bari), Italy Installer: SAEM Company Solar PV: Yingli Inverters: Siel Size: 3 MWp Return: €1 412 000 per year The solar power plant was built by SAEM Company and is made up of 13 500 units. The plant is oriented to the south. The plant produces enough energy to power the homes of 1500 families. 11. Huerto Solar Villar de Cañas IICountry: Villar de Cañas, Spain Installer: CYMI Solar PV: Yingli Inverters: Siemens Size: 9.8 MWp Return: €6 336 000 per year Prosolcam bought a 22 hectare site to invest in solar energy. The company CYMI designed and installed the system that consist of 56 180 pv modules. The plant has an south facing orientation. 12. Amcorp Gemas Solar PlantCountry: Gemas, Negeri Sembilan, Malaysia Installer: Amcorp Power Sdn. Bhd. Solar PV: Yingli Size: 10 269 MWp Return: MYR 11.88 million (about $2.6 million) Amcorp Power is a solar farm developer in Malaysia. The solar plant has a power purchase agree with Tenaga Nasional Berhad for 21 years. The plant that consists of 41 076 pv modules, produces enough energy for 3315 residential homes. Read Case Study 13. Jackson Enterprise LLCCountry: California, United States Installer: CM Solar Electric Solar PV: Sunpower, LG Inverter: SMA Size: 26kW Average Annual Savings: $11 556 Return on Investment: 23.6% The solar energy system provides at least 100% or more of the energy consumption of the building. And the total net investment of the system was $49 000. 14. Diab EngineeringCountry: Geraldton, Australia Installer: Infinite Energy Solar PV: Conergy Inverter: SMA Size: 100 kW Year 1 return on investment: 34% 10 Year Net Present Value: $139 000 Annual Savings: $41 000 Diab Engineering choose Infinite Energy to install a solar energy system on there roof of their workshop. Diab Engineering used government funded solar programmes to finance their system. 15. GAL ManufacturingCountry: New York,United States Installer: Solar City Size: 237 kW Annual Savings: $50 000 GAL Manufacturing is a family owned company that builds elevator parts. The system will generate almost half of the buildings energy consumption. The project is partly funded by government funds. 16. Hewlett PackardCountry: Palo Alto, California, United States Size: 1 MW Estimated Lifetime Savings: $1 million HP installed 1 MW of solar modules on its roof. The system will provide 20% of the buildings usage. HP doesn’t own the system, but will purchase the energy produced from Solar City. 17. Velmade Prestige Sheet MetalCountry: Osborne Park, Australia Installer: Infinite Energy Solar PV: REC Solar Inverter: SMA Size: 31 kW Year 1 return on investment: 18% 10 year Net Present Value: $7 400 Annual Savings: $8 500 In 2014 Velmade installed 120 solar modules on its roof. As a small-to-medium business it wanted to reduce its operating costs. The project is expected to payback in 5.3 years. Velmade used outside funding for its solar system. 18. Bella Ridge WineryCountry: Herne Hill, Australia Installer: Infinite Energy Solar PV: REC Solar Inverter: SMA Size: 40 kW Year 1 return on investment: 21% Annual Savings: $18 300 Bella Ridge Winery is a energy intensive company and was suffering of rising electricity prices in Australia. Infinite Energy installed 156 REC Solar modules on a ground mounted rack. The projected payback period is 4.4 years. 19. Cheeky BrothersCountry: Osborne Park, Australia Installer: Infinite Energy Solar PV: REC Solar Inverter: Fronius Size: 40 kW Year 1 return on investment: 28% Annual Savings: $13 500 Cheeky Brothers is a Food company that installed 152 REC Solar panels on its roof. The system produces 28% of electricity consumption. 20. Seven Acres Business ParkCountry: Suffolk, United Kingdom Installer: Enviko Solar PV: CSUN Inverter: SMA Size: 40 kW Yearly Income and Savings: £8 079 This business park decided to install 120 solar panels on its roof just in time before feed in tariffs were reduced in 2012. The project was completed just in time by Enviko. 21. Broad Oak Cider FarmCountry: Clutton Hill Industrial Park, Bristol, United Kingdom Installer: Enviko Solar PV: Conergy Inverter: Solaredge Size: 100 kW Yearly Income and Savings: £15 894 Enviko helped Broad Oak Cider Farm install 400 solar panels that covered the whole roof of the building. Power optimizers were used to reduce the effects of shading on the panels. 22. Glebar Inc.Country: Franklin Lakes, New Jersey, United States Installer: Solar Energy World Solar PV: Schuco Size: 55.5 kW Yearly Savings: $8000 Glebar Inc was looking for a way to reduce its energy bills and reduce its carbon footprint. Solar Energy World helped achieving their goals. The system is partly funded with a tax break and Solar Renewable Energy Credits. 23. Metuchen SportscomplexCountry: Metuchen, New Jersey, United States Installer: Solar Energy World Solar PV: LG Size: 312 kW Yearly Savings: $33 397 The developer Recycland LLC decid to add Solar Energy to its building to reduce energy costs and to reduce its carbon footprint. 24. Alfandre ArchitectureCountry: New Paltz, New York, United States Installer: Sunrise Solar Solutions Solar PV: Conergy and Hyunday Inverter: Enphase Size: 33.4 kW Savings over lifetime system: $190 000 Alfandre Architecture is applying for the LEED GOLD Certification. Adding solar energy to the project is a logical step. Sunrise Solar Solutions did the installation of the new building. Country: San Jose, California, United States Installer: Solar City Size: 650 kW Annual Cost Savings: $100 000 Ebay wanted to make its campus in San Jose more sustainable. Solar City designed and installed the 3248 solar panel system on five different buildings located on the campus. 26. Heritage PaperCountry: Livermore, California, United States Installer: Solar City Size: 528 kW Annual Cost Savings: $26,950 Heritage Paper is the packaging supplier of big companies like Nordstrom and Cliff Bar. Their huge facility uses huge amounts of energy and installing solar panels was a no-brainer. Read cases study 27. Batth FarmsCountry: San Joaquin Valley, California, United States Installer: Solar City Size: 1.5 MW Estimated lifetime savings: $9 000 000 The Batth farm uses a lot of energy for the irrigation of the land and running waterpumps. To reduce their operating costs Solar City installed a solar energy system on their farmland. 28. Advance Auto PartsCountry: Enfield, Connecticut, United States Installer: Solar City Size: 1.17 MW Annual Cost Savings: $100 000 Advance Auto Parts is a distribution company of after-sales auto parts. Solar City installed the solar system with little to no disruption to daily operations. 29. RoofmartCountry: Kewdale, Australia Installer: Infinite Energy Solar PV: REC Solar Inverter: SMA Size: 100 kW Year 1 Return on Investment: 25% 10 year Net Present Value: $103 200 Annual Savings: $37 600 Roofmart design, manufactures and distributes steel constructions that are used for garages, patios and sheds. The system was installed in december 2015 and the cost will be returned in under 4 years. Country: Osborne, Australia Installer: Infinite Energy Solar PV: Winaico Inverter: SMA Size: 100 kW Year 1 Return on Investment: 32% 10 Net Present Value: $240 300 Annual Savings: $45 300 Imdex is listed on the ASX and produces and manufactures fluids and instruments for the mining, oil and gas industries. The projected payback period the solar energy system will be 3.1 years. 31. Audi SeattleCountry: Seattle, United States Installer: A&R Solar Solar PV: Sunpower Size: 235 kW Estimated 25 year savings: $2 million Audi Seattle is a dealer of high performance electric vehicles. The company wanted to power their vehicles with a sustainable energy source, solar energy. 32. Boulder NissanCountry: Boulder, United States Installer: Independent Power Systems Solar PV: Sunpower Size: 50.25 kW Estimated 25 year savings: $384 000 Boulder Nissan is a high volume seller of the electric Nissan Leaf in the Boulder area. The adoption of solar energy is a logical step. 34. MicrosoftCountry: Mountain View, United States Solar PV: Sunpower Size: 551861 kW Estimated annual savings: $120 000 Microsoft is one of the biggest software companies in the world with a commitment to the environment. 35. Rivermaid Trading co.Country: California, United States Installer: Sunworks Solar PV: Sunpower Size: 1.7 mW Estimated annual savings: $300 000 Rivermaid Trading is a grower, processor and distributer of fruit. The company has facitlities that are huge and with solar energy they wanted to reduce their energy bills. 36. Lake County Sanitation DistrictCountry: Lakepoint, United States Solar PV: Sunpower Size: 2.17 mW Estimated savings over 20 years: $5 million The Lake County Sanitation District wanted to reduce their environmental impact. 37. Dobinsons Spring & SuspensionCountry: Rockhampton, Australia Solar PV: Hanwha Q Cells Size: 510 kWp Estimated annual savings: AUD$160 000 In the past decade Dobinsons saw their energy costs grow with 100%. With an solar energy system Dobinsons is now protected from increasing energy prices. 38. AustchilliCountry: Bundaberg, Australia Solar PV: Phono Solar Size: 300 kWp Estimated payback period of 4-5 years Rising energy costs made the business model of Austchilli less feasible and that is why they choose solar energy. 39. Enmach IndustriesCountry: Bundaberg, Australia Solar PV: Q-Cell Size: 100 kWp Estimated annual savings: AUD$40 000 Estimated payback period of 3.5 years Like a lot of Australian manufacturing companies, the energy bill of Enmach Industries was rising. Solar energy was the only logical solution. 40. Advantage WeldingCountry: Rockhampton, Australia Solar PV: Phono Solar Size: 33 kWp Estimated payback period of 4.2 years To reduce their electricity bill Advantage Welding worked together with Gem Energy to install solar energy panels on their roof. 41. Bridge ToyotaCountry: Darwin, Australia Solar PV: Q Cells Size: 100 kWp Estimated annual savings AUD$35 000 Estimated payback period of 3.5 years Bridge Toyota has a huge energy consumption for its showroom, office, workshop and warehouse. To prevent huge energy bills cutting in their operating margins they switched to a solar energy system on the roof of their facility. 42. Great Western HotelCountry: Rockhampton, Australia Solar PV: Q Cells Size: 57 kWp Estimated payback period of 3.2 years The Great Western Hotel used a renovation to make their operation more green with a solar energy system that is connected to the grid. 43. Luther Auto GroupCountry: Midwest, United States Solar PV: Sunpower Size: 454 kWp Estimated saving over 25 years: $2.1 million The Luther Auto Group used their large flat roofs of their dealerships to generate cheap solar energy. 44. Turtle Bay ResortCountry: Kahuku, United States Solar PV: REC Solar Size: 702 kWp Estimated saving over 20 years: $2.5 million The Turtle Bay Resort won the Leader in Sustainability Award in Hawaii. The Turtle Bay Resort worked together with REC Solar to install a roof mounted system and a ground mounted system. 45. Zurn IndustriesCountry: Paso Robles, United States Solar PV: REC Solar Size: 552.7 kWp Estimated annual savings: $110 000 Zurn Industries is a manufacturer of irrigation equipment and want to reduce their operating expenses with the installation of a roof mounted solar energy system. 46. San Antonio WineryCountry: Paso Robles, United States Solar PV: REC Solar Size: 517 kW Estimated saving over 30 years: $4 million The San Antonio WInery will produce 80% of the power they need for their wine production facility and their hospitality center. 47. Ballester HermanosCountry: San Juan, United States Solar PV: REC Solar Size: 874 kW Estimated annual savings: $100 000 Ballester Hermanos is located on Puerto Rico that has high energy prices. Solar energy through a power purchase agreement made a lot of economic sense. 48. Sonoma Mountain VillageCountry: Rohnert Park, United States Solar PV: REC Solar Size: 1.16 mW Estimated annual savings: $680 000 Sonoma Mountain Village improved their Leed Premium status by expanding their solar energy capacity. 49. Haas Automation Inc.Country: Oxnard, United States Solar PV: REC Solar Size: 1.74 mW Estimated annual savings: $500 000 Haas automation wanted to reduce their carbon footprint and reduce their energy costs and opted for two solar roos systems in partnership with Renusol. 50. Niner Wine EstatesCountry: Paso Robles, United States Solar PV: REC Solar Size: 388.47 kW Estimated payback period of 5 years Niner Wine Estates is a Sustainability in Practice Certified winery and has an LEED status. Through their solar energy system they generate 100% of their energy needs. 51. Valley Fine FoodsCountry: Benecia and Yuba City, United States Solar PV: REC Solar Size: 1.14 mW Estimated annual savings: $250 000 Valley Fine Foods used a roof mounted and ground mounted solar system to reduce their energy cost. 52. Tony Automotive GroupCountry: Waipahu, United States Solar PV: REC Solar Size: 298 kW Estimated savings over 25 years: $5.3 million Tony Automotive groups has Honda, Nissan and Hyundai dealerships in Hawaii. The need for solar energy was great, because Hawaii has the highest energy costs in the nation. 53. Windset FarmsCountry: Santa Maria, United States Solar PV: REC Solar Size: 1.05 mW Estimated annual savings: $245 000 The Windset Farms installed more than 4000 solar energy panels on their roof to curb their rising energy bill. 54. Vintage Wine EstatesCountry: Santa Rosa & Hopland, United States Solar PV: REC Solar Size: 945 kW Estimated savings over 30 year period: $10 million Vintage Wine Estates used a combination of roof mounted and ground mounted solar panels to reduce their utility costs. Country: Bibra Lake, United States Solar PV: Conenergy Size: 350 kW Estimated annual savings: AUD$169 000 AWTA is the largest wool testing organization in the world. The installed 1085 solar panels on their roof and produce 32% of their energy consumption. 56. TransminCountry: Malaga, Australia Solar PV: Suntech Size: 40 kW Estimated annual savings: AUD$15 200 With the help of the AusIndustry Clean Technology Investment Program, Transmin made their operations more sustainable with 174 Suntech panels and 2 SMA solar inverters. 57. Mining & Hydraulic Supplies Pty LtdCountry: Malaga, Australia Solar PV: Solarpower Size: 7 kW Estimated annual savings: AUD$1900 Mining & Hydraulic Supplies has reduced their electricity bill significantly and generate 80% of their energy with solar panels. 58. T&G CorporationCountry: Perth, Australia Solar PV: Suntech Size: 33 kW Estimated annual savings: AUD$9800 In the preceding years T&G Corporation saw their utility bills rise 28%. With solar energy the made their future energy bills predictable again. 59. Firesafe United GroupCountry: Bibra Lake, Australia Solar PV: Hanwha Size: 80 kW Estimated annual savings: AUD$23 500 Firesafe United Group installed 3 solar energy systems on their roof to optimize their energy costs. 60. Pacific Nylon Plastics AustraliaCountry: O’Connor, Australia Solar PV: Canadian Solar Size: 20 kW Estimated annual savings: AUD$10 700 Pacific Nylon Plastics Australia used the redevelopment of their buildings to make their operations greener with the installation of 80 solar pv panels 61. Sheridan’sCountry: West Perth, Australia Solar PV: Daqo Size: 15 kW Estimated annual savings: AUD$6 100 Sheridan’s installed with their installation partner Infinity Energy 60 solar panels on their roof and one fronius solar inverter. 62. Signs & LinesCountry: Midvale, Australia Solar PV: Q Cells Size: 40 kW Estimated annual savings: AUD$13 500 Cost control was a major reason for Sign & Lines to choose for a roof mounted solar energy system. 63. SlumbercorpCountry: Welshpool, Australia Solar PV: REC Solar Size: 40 kW Estimated annual savings: AUD$16 100 64. WA GlasskoteCountry: Landsdale, Australia Solar PV: REC Solar Size: 40 kW Estimated annual savings: AUD$10 200 WA Glasskote generates 12% of its energy consumption with their solar energy system. Country: Malaga, Australia Solar PV: REC Solar Size: 200 kW Estimated annual savings: AUD$82 854 Dobbie wanted to reduce their impact on the environment and their energy costs. Country: Belmont, Australia Solar PV: REC Solar Size: 30 kW Estimated annual savings: AUD$15 100 Pindan, a construction company, generates 7% of their energy usage with solar panels. 67. Wallis DrillingCountry: Midvale, Australia Solar PV: REC Solar Size: 67 kW Estimated annual savings: AUD$28 900 Wallis Drilling wanted to reduce their costs and make their operations more sustainable. They choose for a roof mounted solar energy system with four Fronius solar inverters. Their solar energy electricity consumption represents 47% of their total energy consumption. 68. GeostatsCountry: O’Connor, Australia Solar PV: REC Solar Size: 20 kW Estimated annual savings: AUD$6 600 Geostats wanted to make their operations more environmentally friendly and optimize their energy costs. 69. Eilbeck CranesCountry: Bassendean, Australia Solar PV: Canadian Solar Size: 40 kW Estimated annual savings: AUD$15 800 Eilbeck Cranes installed 156 Canadian Solar on their roof connected to two Fronius inverters monitored with Fronius Remote Monitoring Solution. 70. ArbortechCountry: Malaga, Australia Solar PV: Poly Solar Panels Size: 40 kW Estimated annual savings: AUD$13 000 Arbortech wanted to reduce its dependency on the utility prices by switching to rooftop solar. 71. Australian Safety EngineersCountry: Canning Vale, Australia Solar PV: REC Solar Size: 40 kW Estimated annual savings: AUD$22 100 Australian Safety Engineers wanted to decrease their utility bill. They opted for a rooftop solar energy system. 72. StylewoodsCountry: Kewdale, Australia Solar PV: Winaico Solar Panels Size: 40 kW Estimated annual savings: AUD$31 500 Stylewoods wanted to reduce their energy bill to free up more working capital for their operations. 73. Plas-PakCountry: Malaga, Australia Solar PV: Winaico Solar Panels Size: 100 kW Estimated annual savings: AUD$31 500 Plas-Pak wanted to maintain competitive prices for their clients and to make their company more environmentally friendly. 74. John Papas TrailersCountry: Welshpool, Australia Solar PV: REC Solar Size: 40 kW Estimated annual savings: AUD$13 300 John Papas Trailers reduced their dependence on grid electricity through the decision for a solar energy system. 75. Quality Blast and PaintCountry: Welshpool, Australia Solar PV: Sunpower Size: 40 kW Estimated annual savings: AUD$12 550 Quality Blast and Paint wanted to become more competitive through the adoption of solar energy. 76. Pelagic Marine ServicesCountry: Freemantle, Australia Solar PV: Sunpower Size: 40 kW Estimated annual savings: AUD$15 530 Pelagic Marine Services wanted to make their business more sustainable and more cost efficient and choose for a solar energy system installed by Infinity Energy. 77. Twenty Two ServicesCountry: Neerabup, Australia Solar PV: Sunpower Size: 13 kW Estimated annual savings: AUD$4 200 Twenty Two Services wanted to reduce their yearly CO2 emissions and their utility bills. Infinity Energy helped them install solar energy system containing 38 solar panels and one Fronius inverter. 78. Yolo CountyCountry: California, United States Solar PV: Sunpower Size: 6.8 mW Estimated savings over 30 years: $60 million Yolo county wanted to reduce their energy bill and supply their residents with green energy. 79. AC Transit DistrictCountry: California, United States Installer: Sunpower Size: 177 kW Estimated savings over 25 years: $5 million ACT Transit District is is Sunpower helped AC Transit District with the installation of two solar energy projects. 80. US Airforce AcademyCountry: Colorado Springs, United States Solar PV: Sunpower Size: 6 mW Estimated savings annual savings: $500 000 81. Department of Mines and PetroleumCountry: Carlisle, Australia Installer: Infinite Energy Solar PV: Winaico Inverter: SMA Size: 40 kW Year 1 Return on Investment: 31% 10 Year Net Present Value: $69 200 Annual Savings: $15 400 Infinite Energy installed 153 solar panels on the roof of the Department of Mines and Petroleum. The projected return is 2.8 years. 82. Sacred Hearts AcademyCountry: Hawaii, United States Installer: Hawaiian Energy Systems Solar PV: Centrosolar America Solar Inverter: Enphase Size: 243 kW Cost Reduction: 33% annually Sacred Hearts Academy is a private school in Honolulu, Hawaii. Hawaiian Energy Systems inc. and Centrosolar America installed 1023 panels on three different sun orientations and was completed in 2013. 83. Ina Levine Jewish Community CenterCountry: Arizona, United States Installer: Green Choice Solar Solar PV: Centrosolar America Size: 1.3 MW Cost reduction: $6.8 million lifetime system The Ina Levine Jewish Community Center delivers services to the Scottsdale community. Green Choice Solar installed 5685 solar panels on two locations. One part of the panels was installed on the roof and the majority was installed on 400 carports. 84. Fire station GifhornCountry: Germany Installer: Elektro Ohlhoff Solar PV: Yingli Solar Inverter: Kaco Powador Size: 60.86 kWp Cost Reduction: €25900 The roofs of the fire station in Gifhorn presented a perfect solar energy investment opportunity. It was an easy decision for the local government of Gifhorn. 85. University of ColoradoCountry: Boulder, Colorado, United States Installer: Eco Depot USA / Solarado Energy Inverter: SatCon Technology Corporation Size: 100 kW Average Annual Savings: $21 750 Return on investment: 7.9% In septembre 2009 the University of Colorado installed solar panels on a solar carport. This project was part of a LEED Platinum certificate process for which the University applied. The LEED platinum status is the highest green building status that can be achieved in the LEED program. 86. Rotary Residential CollegeCountry: Kensington, Australia Installer: Infinite Energy Solar PV: REC Solar Inverter: SMA Size: 40 kW Year 1 return on investment: 33% 10 year Net Present Value: $69 000 Annual Savings: $20 400 Rotary Residential College is a high-school with a lodging service to their students. Infinite Energy helped the Rotary Residential College with the installation of 153 REC solar panels on their roof. 87. Solar Carport Santa CruzCountry: Santa Cruz, California, United States Installer: Swenson Solar Size: 386 kW Annual Savings: $73 000 The city of Santa Cruz choose Swenson Solar to build two solar carports with 834 and 936 solar panels installed on them. 88. Hurstpierpoint CollegeCountry: Hurstpierpoint, United Kingdom Installer: Enviko Solar PV: Conergy Inverter: SMA Size: 53.75 kW Yearly Income and Savings: £10 151 Hurstpierpoint is a college home to more than 1000 students. The college wanted to reduce their energy bill and demonstrate their green credentials. The solar panels are installed on three different roofs. Because of the feed-in-tariff the cost of the installation will be recovered in 6 years. 89. San Ramon Valley Unified School DistrictCountry: Danville, United States Solar PV: Sunpower Size: 3.3 mW Estimated savings over 25 years: $24.4 million The San Ramon Valley Unified School District was confronted with the reduction of their budgets and growing energy bills. Getting solar energy was their solution. 90. University of California MercedCountry: Merced, United States Solar PV: Sunpower Size: 1.1 mW Estimated savings over 20 years: $5 million The university wanted to reach their sustainable goals and with no upfront cost the adopted solar energy through a power purchase agreement. 91. Stonehill CollegeCountry: Easton, United States Solar PV: Sunpower Size: 2.8 mW Estimated savings over 20 years: $1.8 million The Stonehill College started the Stonehill Goes Green campaign to reduce their gas emmission with 20% by 2020. That is why they switched to solar energy paid through a power purchase agreement. 92. Inland Empire Utilities AgencyCountry: San Bernardino County, United States Solar PV: Sunpower Size: 3.5 mW Estimated savings over 20 years: $3 million The Inland Empire Utilities Agency has the objective to be 100% powered by renewable energy by 2020. 93. Phelan Piñon Hills Community Services DistrictCountry: San Bernardino County, United States Solar PV: Sunpower Size: 1.5 mW Estimated savings over 30 years: $13 million The Phelan Piñon Hills Community Services District was confrented with fast growing electricity prices and lowered their cost with solar energy. 94. Bundaberg Christian CollegeCountry: Bundaberg, Australia Solar PV: Hanwha Q Cells Size: 193.98 kWp Estimated annual savings: AUD$100 000 The Bundaberg Christian College has opted for a solar energy system with battery backup, the largest system of its kind at an Australian school. 95. Cathedral CollegeCountry: Rockhampton, Australia Solar PV: Q Cells Size: 85 kWp Payback period of six years Because of it strong commitment to sustainability, Cathedral College opted for solar energy. 96. Emerald Marist CollegeCountry: Central Highlands, Australia Solar PV: Q Cells Size: 100 kWp Estimated annual savings: AUD$40 000 Due to high air conditioning usage and electricity bills during the summer months, Emerald Marist College, choose to install a solar energy system on its roof. 97. Pleasanton Unified School DistrictCountry: Paso Robles, United States Solar PV: REC Solar Size: 1 mW Estimated saving over 25 years: $2.2 million The Pleasanton Unified School District made the switch to solar energy through a power purchase agreement. The solar panels were placed on solar carports. 98. Roseville Joint Union High School DistrictCountry: Paso Robles, United States Solar PV: REC Solar Size: 1.02 mW Estimated saving over 25 years: $8 million The Roseville Joint Union High School District installed solar panels over their parking structures. 99. St Catherine’s CollegeCountry: Crawley, Australia Solar PV: Sunpower Size: 200 kW Estimated annual savings: AUD$84 000 100. City of Perth – DepotCountry: Perth, Australia Solar PV: Sunpower Size: 39 kW Estimated annual savings: AUD$16 100 The city of Perth wanted to make their depot more sustainable and more cost efficient. Pin It on Pinterest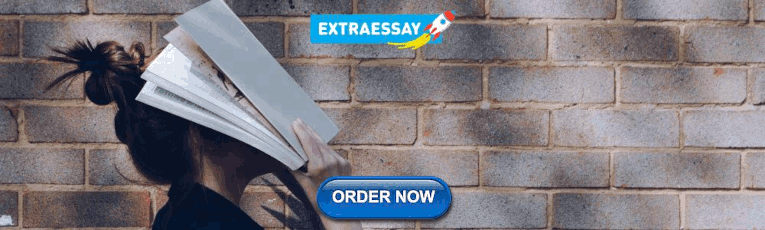 |
COMMENTS
Case Study 8 - Photovoltaic Cells. In 2009 the total installed capacity of solar PV in the United States was 1.64 GW. In 2019, the U.S. installed 2.7 gigawatts (GW) of solar PV capacity in the first quarter of the year to reach 67 GW of total installed capacity, enough to power 12.7 million American homes. Use the formula below to calculate the ...
Study with Quizlet and memorize flashcards containing terms like A photovoltaic cell or device converts sunlight to ___, PV systems operating in parallel with the electric utility system are commonly referred to as ___ systems, PV systems operating independently of other power systems are commonly referred to as ___ systems and more.
Activity 1: Solar cell(s) and small electric fan. Attach a solar cell, or multiple solar cells wired in series or parallel or any combination thereof, to a small electric motor with a fan blade attached. ***Be careful not to injure yourself with the fan blade. High RPMs can be achieved even with these small motors.***.
1839: Photovoltaic Effect Discovered: Becquerel's initial discovery is serendipitous; he is only 19 years old when he observes the photovoltaic effect. 1883: First Solar Cell: Fritts' solar cell, made of selenium and gold, boasts an efficiency of only 1-2%, yet it marks the birth of practical solar technology. 1905: Einstein's Photoelectric Effect: Einstein's explanation of the ...
Study with Quizlet and memorize flashcards containing terms like ATP and photovoltaic cells are similar because, Which molecule is a high-energy output of the light reactions?, In photosynthesis, light energy is and more. ... The is the practice study cards of the cells and what's inside the cell. 8 terms. Alivia_Cosby. Preview. Cells / Cell ...
K. Mertens Textbook PV Solutions to Exercises Solutions to Chapter 1: Exercise 1.1: Energy Content a) W = 1 kg 8.14 kWh/kg = 8.14 kWh W = 8.14 kWh = 8140 W 3.600 s = 29 304 000 Ws = 29.304 MJ b) 2987 km 1 kg 9.81 m/s 29.304 10 J 2 6 Pot Pot m g W W m g h h 3000 km c) 2 Kin 2 1 W m v m W v Kin 2 = 1000 kg
Answer Key - Chapter 25 (31.0K) Answer Key - Chapter 26 (36.0K) To learn more about the book this website supports, please visit its Information Center .
5.1.2 Electricity Generation with Solar Cells The photovoltaic effect is the basic physical process through which a PV cell converts sunlight into electricity. Sunlight is composed of photons (like energy accumulations), or particles of solar energy. These photons contain various amounts of energy
The best panels for commercial use have efficiencies around 18% to 22%, but researchers are studying how to improve efficiency and energy yield while keeping production costs low. Read more about solar PV research directions in Part 2! Part 1 of the PV Cells 101 primer explains how a solar cell turns sunlight into electricity and why silicon is ...
Nearly all types of solar photovoltaic cells and technologies have developed dramatically, especially in the past 5 years. Here, we critically compare the different types of photovoltaic ...
Photovoltaic Cell: Photovoltaic cells consist of two or more layers of semiconductors with one layer containing positive charge and the other negative charge lined adjacent to each other. Sunlight, consisting of small packets of energy termed as photons, strikes the cell, where it is either reflected, transmitted or absorbed.
Employing sunlight to produce electrical energy has been demonstrated to be one of the most promising solutions to the world's energy crisis. The device to convert solar energy to electrical energy, a solar cell, must be reliable and cost-effective to compete with traditional resources. This paper reviews many basics of photovoltaic (PV) cells, such as the working principle of the PV cell ...
also a chapter on advanced types of silicon cells. Chapters 6-8cover the designs of systems constructed from individual cells-includingpossible constructions for putting cells together and the equipment needed for a practioal producer of electrical energy. In addition, Chapter 9 deals with PV'sfuture. Chapter 1 is a general introduction to the ...
Figure 1 Price evolution (from factories) (blue) for PV modules and total yearly world production (red) of PV solar cells (logarithmic scale); the prices are in current dollars per 1-W peak power rating ($/Wp) (blue). If corrected for inflation, the price decrease between 1975 and 1985 is much steeper; the projection after 1998 is based on maintaining the same cost reduction rate of 7.5% as ...
The global weighted average LCOE of utility-scale PV plants is estimated to have fallen by 77% between 2010 and 2018, from around USD .37/kWh to USD 0.085/ kWh, while auction and tender results suggest they will fall to between USD .08/kWh and .02/kWh in 2030.
Over the past decade, the solar installation industry has experienced an average annual growth rate of 24%.A 2021 study by the National Renewable Energy Laboratory (NREL) projected that 40% of all power generation in the U.S. could come from solar by 2035.. Solar's current trends and forecasts look promising, with photovoltaic (PV) installations playing a major role in solving energy ...
case study and reference document detailing the steps and processes that could be used to successfully identify, fund, and implement solar photovoltaics (PV) projects in school districts across the country. Keywords: schools; solar photovoltaics . 1. School districts across the United States face trying times
This case study describes what is, and how a solar cell works; ... This case study describes what is, and how a solar cell works; it is a semiconductor device that converts the energy of. Post a Question. Provide details on what you need help with along with a budget and time limit. ...
The adoption of solar energy is a logical step. Read case study. 34. Microsoft. Country: Mountain View, United States. Solar PV: Sunpower. Size: 551861 kW. Estimated annual savings: $120 000. Microsoft is one of the biggest software companies in the world with a commitment to the environment.
Abstract and Figures. The building integrated photovoltaic (BIPV) system have recently drawn interest and have demonstrated high potential to assist building owners supply both thermal and ...
Introduction and Agenda. Session Objectives: Provide update on the Better Buildings Alliance's Renewables Integration Team. Present case studies and strategies from successful solar PV projects on commercial buildings. Agenda. Introduction - Jay Paidipati. A Commercial Building Owner Perspective - Eugenia. 2.