NOTIFICATIONS
Mendel’s experiments.
- + Create new collection
Mendel is known as the father of genetics because of his ground-breaking work on inheritance in pea plants 150 years ago.
Gregor Johann Mendel was a monk and teacher with interests in astronomy and plant breeding. He was born in 1822, and at 21, he joined a monastery in Brünn (now in the Czech Republic). The monastery had a botanical garden and library and was a centre for science, religion and culture . In 1856, Mendel began a series of experiments at the monastery to find out how traits are passed from generation to generation. At the time, it was thought that parents’ traits were blended together in their progeny .
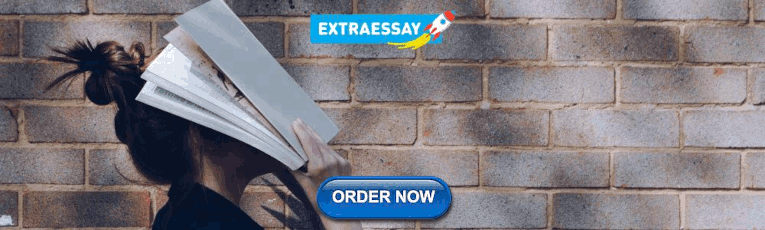
Studying traits in peas
Mendel studied inheritance in peas ( Pisum sativum ). He chose peas because they had been used for similar studies, are easy to grow and can be sown each year. Pea flowers contain both male and female parts, called stamen and stigma , and usually self-pollinate. Self-pollination happens before the flowers open, so progeny are produced from a single plant.
Peas can also be cross-pollinated by hand, simply by opening the flower buds to remove their pollen-producing stamen (and prevent self-pollination) and dusting pollen from one plant onto the stigma of another.
Traits in pea plants
Mendel followed the inheritance of 7 traits in pea plants, and each trait had 2 forms. He identified pure-breeding pea plants that consistently showed 1 form of a trait after generations of self-pollination.
Mendel then crossed these pure-breeding lines of plants and recorded the traits of the hybrid progeny. He found that all of the first-generation (F1) hybrids looked like 1 of the parent plants. For example, all the progeny of a purple and white flower cross were purple (not pink, as blending would have predicted). However, when he allowed the hybrid plants to self-pollinate, the hidden traits would reappear in the second-generation (F2) hybrid plants.
Dominant and recessive traits
Mendel described each of the trait variants as dominant or recessive Dominant traits, like purple flower colour, appeared in the F1 hybrids, whereas recessive traits, like white flower colour, did not.
Mendel did thousands of cross-breeding experiments. His key finding was that there were 3 times as many dominant as recessive traits in F2 pea plants (3:1 ratio).
Traits are inherited independently
Mendel also experimented to see what would happen if plants with 2 or more pure-bred traits were cross-bred. He found that each trait was inherited independently of the other and produced its own 3:1 ratio. This is the principle of independent assortment.
Find out more about Mendel’s principles of inheritance .
The next generations
Mendel didn’t stop there – he continued to allow the peas to self-pollinate over several years whilst meticulously recording the characteristics of the progeny. He may have grown as many as 30,000 pea plants over 7 years.
Mendel’s findings were ignored
In 1866, Mendel published the paper Experiments in plant hybridisation ( Versuche über plflanzenhybriden ). In it, he proposed that heredity is the result of each parent passing along 1 factor for every trait. If the factor is dominant , it will be expressed in the progeny. If the factor is recessive, it will not show up but will continue to be passed along to the next generation. Each factor works independently from the others, and they do not blend.
The science community ignored the paper, possibly because it was ahead of the ideas of heredity and variation accepted at the time. In the early 1900s, 3 plant biologists finally acknowledged Mendel’s work. Unfortunately, Mendel was not around to receive the recognition as he had died in 1884.
Useful links
Download a translated version of Mendel’s 1866 paper Experiments in plant hybridisation from Electronic Scholarly Publishing.
This apple cross-pollination video shows scientists at Plant & Food Research cross-pollinating apple plants.
See our newsletters here .
Would you like to take a short survey?
This survey will open in a new tab and you can fill it out after your visit to the site.
- Biology Article
- Mendel Laws Of Inheritance
Mendel's Laws of Inheritance
Inheritance can be defined as the process of how a child receives genetic information from the parent. The whole process of heredity is dependent upon inheritance and it is the reason that the offsprings are similar to the parents. This simply means that due to inheritance, the members of the same family possess similar characteristics.
It was only during the mid 19th century that people started to understand inheritance in a proper way. This understanding of inheritance was made possible by a scientist named Gregor Mendel, who formulated certain laws to understand inheritance known as Mendel’s laws of inheritance.
Table of Contents
Mendel’s Laws of Inheritance
Why was pea plant selected for mendel’s experiments, mendel’s experiments, conclusions from mendel’s experiments, mendel’s laws, key points on mendel’s laws.
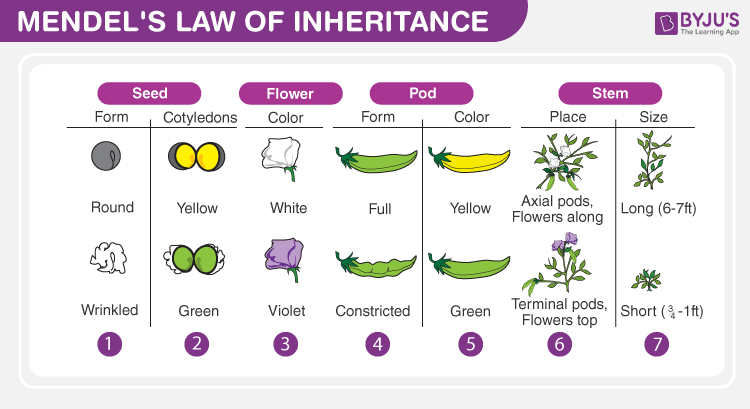
Between 1856-1863, Mendel conducted the hybridization experiments on the garden peas. During that period, he chose some distinct characteristics of the peas and conducted some cross-pollination/ artificial pollination on the pea lines that showed stable trait inheritance and underwent continuous self-pollination. Such pea lines are called true-breeding pea lines.
Also Refer: Mendel’s Laws of Inheritance: Mendel’s Contribution
He selected a pea plant for his experiments for the following reasons:
- The pea plant can be easily grown and maintained.
- They are naturally self-pollinating but can also be cross-pollinated.
- It is an annual plant, therefore, many generations can be studied within a short period of time.
- It has several contrasting characters.
Mendel conducted 2 main experiments to determine the laws of inheritance. These experiments were:
Monohybrid Cross
Dihybrid cross.
While experimenting, Mendel found that certain factors were always being transferred down to the offspring in a stable way. Those factors are now called genes i.e. genes can be called the units of inheritance.
Mendel experimented on a pea plant and considered 7 main contrasting traits in the plants. Then, he conducted both experiments to determine the inheritance laws. A brief explanation of the two experiments is given below.
In this experiment, Mendel took two pea plants of opposite traits (one short and one tall) and crossed them. He found the first generation offspring were tall and called it F1 progeny. Then he crossed F1 progeny and obtained both tall and short plants in the ratio 3:1. To know more about this experiment, visit Monohybrid Cross – Inheritance Of One Gene .
Mendel even conducted this experiment with other contrasting traits like green peas vs yellow peas, round vs wrinkled, etc. In all the cases, he found that the results were similar. From this, he formulated the laws of Segregation And Dominance .
In a dihybrid cross experiment, Mendel considered two traits, each having two alleles. He crossed wrinkled-green seed and round-yellow seeds and observed that all the first generation progeny (F1 progeny) were round-yellow. This meant that dominant traits were the round shape and yellow colour.
He then self-pollinated the F1 progeny and obtained 4 different traits: round-yellow, round-green, wrinkled-yellow, and wrinkled-green seeds in the ratio 9:3:3:1.
Check Dihybrid Cross and Inheritance of Two Genes to know more about this cross.
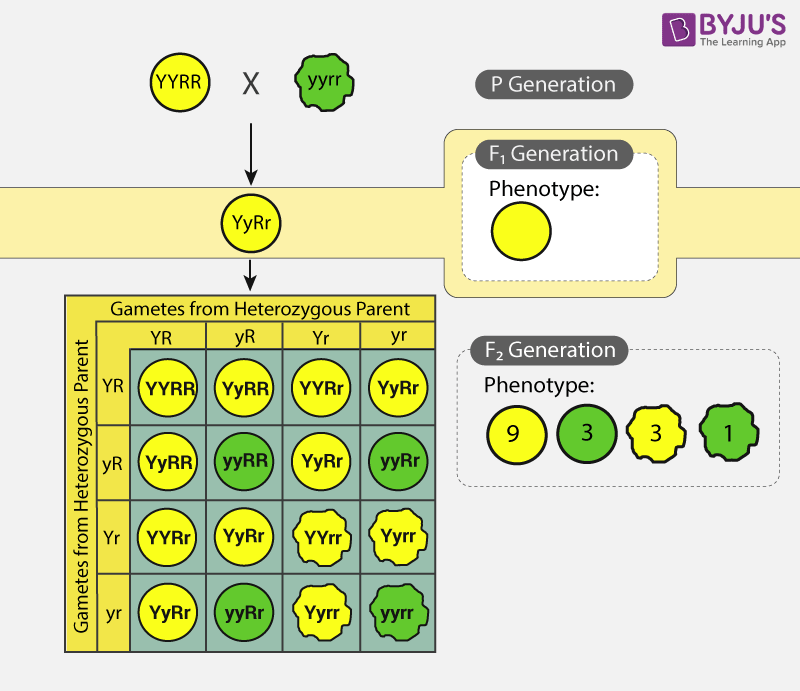
After conducting research for other traits, the results were found to be similar. From this experiment, Mendel formulated his second law of inheritance i.e. law of Independent Assortment.
- The genetic makeup of the plant is known as the genotype. On the contrary, the physical appearance of the plant is known as phenotype.
- The genes are transferred from parents to the offspring in pairs known as alleles.
- During gametogenesis when the chromosomes are halved, there is a 50% chance of one of the two alleles to fuse with the allele of the gamete of the other parent.
- When the alleles are the same, they are known as homozygous alleles and when the alleles are different they are known as heterozygous alleles.
Also Refer: Mendelian Genetics
The two experiments lead to the formulation of Mendel’s laws known as laws of inheritance which are:
- Law of Dominance
- Law of Segregation
- Law of Independent Assortment
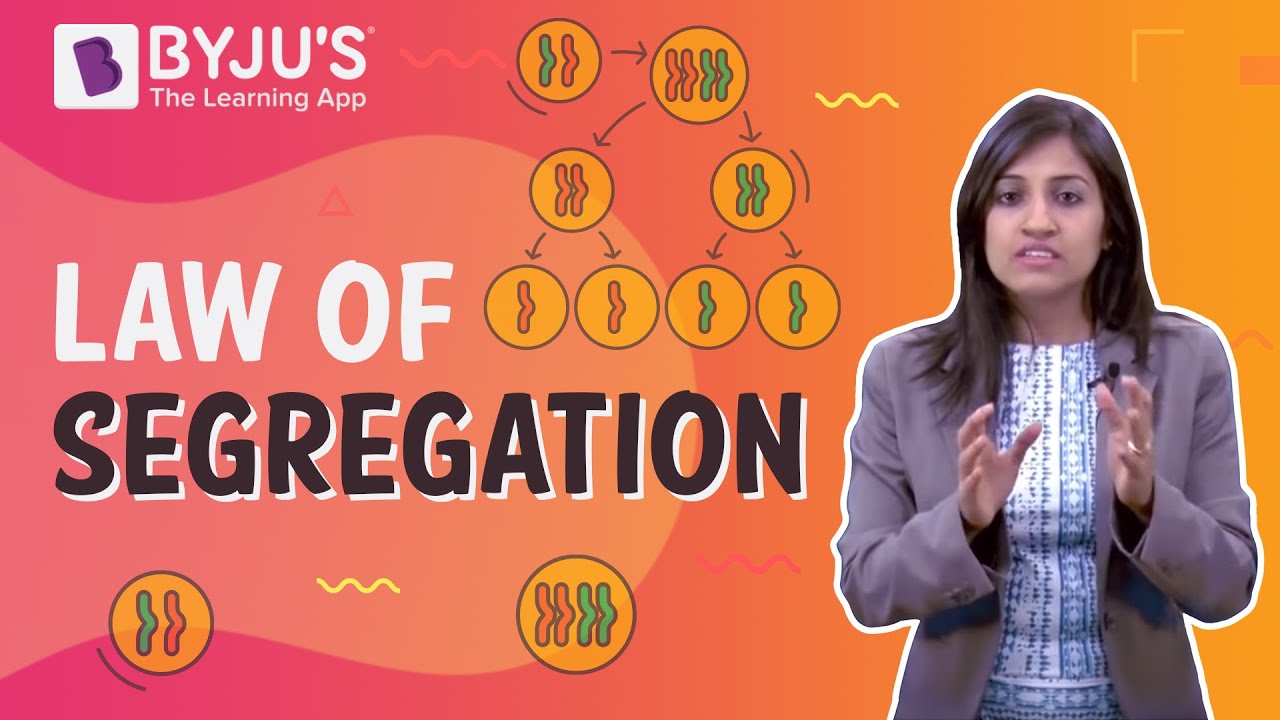
This is also called Mendel’s first law of inheritance. According to the law of dominance, hybrid offspring will only inherit the dominant trait in the phenotype. The alleles that are suppressed are called the recessive traits while the alleles that determine the trait are known as the dominant traits.
The law of segregation states that during the production of gametes, two copies of each hereditary factor segregate so that offspring acquire one factor from each parent. In other words, allele (alternative form of the gene) pairs segregate during the formation of gamete and re-unite randomly during fertilization. This is also known as Mendel’s third law of inheritance.
Also known as Mendel’s second law of inheritance, the law of independent assortment states that a pair of traits segregates independently of another pair during gamete formation. As the individual heredity factors assort independently, different traits get equal opportunity to occur together.
- The law of inheritance was proposed by Gregor Mendel after conducting experiments on pea plants for seven years.
- Mendel’s laws of inheritance include law of dominance, law of segregation and law of independent assortment.
- The law of segregation states that every individual possesses two alleles and only one allele is passed on to the offspring.
- The law of independent assortment states that the inheritance of one pair of genes is independent of inheritance of another pair.
Also Read: Non-Mendelian Inheritance
Stay tuned with BYJU’S to learn more about Mendel’s Laws of Inheritance. You can also download the BYJU’S app for further reference on Mendel’s laws.
Frequently Asked Questions
What are the three laws of inheritance proposed by mendel.
The three laws of inheritance proposed by Mendel include:
Which is the universally accepted law of inheritance?
Law of segregation is the universally accepted law of inheritance. It is the only law without any exceptions. It states that each trait consists of two alleles which segregate during the formation of gametes and one allele from each parent combines during fertilization.
Why is the law of segregation known as the law of purity of gametes?
The law of segregation is known as the law of purity of gametes because a gamete carries only a recessive or a dominant allele but not both the alleles.
Why was the pea plant used in Mendel’s experiments?
Mendel picked pea plants in his experiments because the pea plant has different observable traits. It can be grown easily in large numbers and its reproduction can be manipulated. Also, pea has both male and female reproductive organs, so they can self-pollinate as well as cross-pollinate.
What was the main aim of Mendel’s experiments?
The main aim of Mendel’s experiments was:
- To determine whether the traits would always be recessive.
- Whether traits affect each other as they are inherited.
- Whether traits could be transformed by DNA.
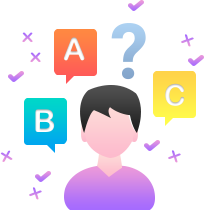
Put your understanding of this concept to test by answering a few MCQs. Click ‘Start Quiz’ to begin!
Select the correct answer and click on the “Finish” button Check your score and answers at the end of the quiz
Visit BYJU’S for all Biology related queries and study materials
Your result is as below
Request OTP on Voice Call
Leave a Comment Cancel reply
Your Mobile number and Email id will not be published. Required fields are marked *
Post My Comment

very nice. it is the best to study about genetics
Genetic inheritance is so interesting
It helped me a lot Thanks
It is so amazing thanks a lot
Superb, it’s interesting.
It is very useful becoz all details explain in simple manner with examples
AWESOME, the above notes are fabulous
well that helped me a lot
Thanks, It helped me a lot!! Impeccable notes !!😍
Nice resource 👍
It helped me alot
If Mendel gave three law the what is the law of unit of characters and who proposed this law . Please clear my doubt a little bit faster , it is little important for me.
The Law of unit characters was proposed by Mendel. He explained that the inheritance of a trait is controlled by unit characters or factors, which are passed from parents to offspring through the gametes. These factors are now known as genes. Each factor exists in pairs, which are known as alleles.

Register with BYJU'S & Download Free PDFs
Register with byju's & watch live videos.
This page has been archived and is no longer updated
Gregor Mendel and the Principles of Inheritance

Traits are passed down in families in different patterns. Pedigrees can illustrate these patterns by following the history of specific characteristics, or phenotypes, as they appear in a family. For example, the pedigree in Figure 1 shows a family in which a grandmother (generation I) has passed down a characteristic (shown in solid red) through the family tree. The inheritance pattern of this characteristic is considered dominant , because it is observable in every generation. Thus, every individual who carries the genetic code for this characteristic will show evidence of the characteristic. In contrast, Figure 2 shows a different pattern of inheritance, in which a characteristic disappears in one generation, only to reappear in a subsequent one. This pattern of inheritance, in which the parents do not show the phenotype but some of the children do, is considered recessive . But where did our knowledge of dominance and recessivity first come from?
Gregor Mendel’s Courage and Persistence
Mendel was curious about how traits were transferred from one generation to the next, so he set out to understand the principles of heredity in the mid-1860s. Peas were a good model system, because he could easily control their fertilization by transferring pollen with a small paintbrush. This pollen could come from the same flower (self-fertilization), or it could come from another plant's flowers (cross-fertilization). First, Mendel observed plant forms and their offspring for two years as they self-fertilized, or "selfed," and ensured that their outward, measurable characteristics remained constant in each generation. During this time, Mendel observed seven different characteristics in the pea plants, and each of these characteristics had two forms (Figure 3). The characteristics included height (tall or short), pod shape (inflated or constricted), seed shape (smooth or winkled), pea color (green or yellow), and so on. In the years Mendel spent letting the plants self, he verified the purity of his plants by confirming, for example, that tall plants had only tall children and grandchildren and so forth. Because the seven pea plant characteristics tracked by Mendel were consistent in generation after generation of self-fertilization, these parental lines of peas could be considered pure-breeders (or, in modern terminology, homozygous for the traits of interest). Mendel and his assistants eventually developed 22 varieties of pea plants with combinations of these consistent characteristics.
Mendel not only crossed pure-breeding parents, but he also crossed hybrid generations and crossed the hybrid progeny back to both parental lines. These crosses (which, in modern terminology, are referred to as F 1 , F 1 reciprocal, F 2 , B 1 , and B 2 ) are the classic crosses to generate genetically hybrid generations.
Understanding Dominant Traits
Understanding recessive traits.
When conducting his experiments, Mendel designated the two pure-breeding parental generations involved in a particular cross as P 1 and P 2 , and he then denoted the progeny resulting from the crossing as the filial, or F 1 , generation. Although the plants of the F 1 generation looked like one parent of the P generation, they were actually hybrids of two different parent plants. Upon observing the uniformity of the F 1 generation, Mendel wondered whether the F 1 generation could still possess the nondominant traits of the other parent in some hidden way.
To understand whether traits were hidden in the F 1 generation, Mendel returned to the method of self-fertilization. Here, he created an F 2 generation by letting an F 1 pea plant self-fertilize (F 1 x F 1 ). This way, he knew he was crossing two plants of the exact same genotype . This technique, which involves looking at a single trait, is today called a monohybrid cross . The resulting F 2 generation had seeds that were either round or wrinkled. Figure 4 shows an example of Mendel's data.
When looking at the figure, notice that for each F 1 plant, the self-fertilization resulted in more round than wrinkled seeds among the F 2 progeny. These results illustrate several important aspects of scientific data:
- Multiple trials are necessary to see patterns in experimental data.
- There is a lot of variation in the measurements of one experiment.
- A large sample size, or "N," is required to make any quantitative comparisons or conclusions.
In Figure 4, the result of Experiment 1 shows that the single characteristic of seed shape was expressed in two different forms in the F 2 generation: either round or wrinkled. Also, when Mendel averaged the relative proportion of round and wrinkled seeds across all F 2 progeny sets, he found that round was consistently three times more frequent than wrinkled. This 3:1 proportion resulting from F 1 x F 1 crosses suggested there was a hidden recessive form of the trait. Mendel recognized that this recessive trait was carried down to the F 2 generation from the earlier P generation .
Mendel and Alleles
As mentioned, Mendel's data did not support the ideas about trait blending that were popular among the biologists of his time. As there were never any semi-wrinkled seeds or greenish-yellow seeds, for example, in the F 2 generation, Mendel concluded that blending should not be the expected outcome of parental trait combinations. Mendel instead hypothesized that each parent contributes some particulate matter to the offspring. He called this heritable substance "elementen." (Remember, in 1865, Mendel did not know about DNA or genes.) Indeed, for each of the traits he examined, Mendel focused on how the elementen that determined that trait was distributed among progeny. We now know that a single gene controls seed form, while another controls color, and so on, and that elementen is actually the assembly of physical genes located on chromosomes. Multiple forms of those genes, known as alleles , represent the different traits. For example, one allele results in round seeds, and another allele specifies wrinkled seeds.
One of the most impressive things about Mendel's thinking lies in the notation that he used to represent his data. Mendel's notation of a capital and a lowercase letter ( Aa ) for the hybrid genotype actually represented what we now know as the two alleles of one gene : A and a . Moreover, as previously mentioned, in all cases, Mendel saw approximately a 3:1 ratio of one phenotype to another. When one parent carried all the dominant traits ( AA ), the F 1 hybrids were "indistinguishable" from that parent. However, even though these F 1 plants had the same phenotype as the dominant P 1 parents, they possessed a hybrid genotype ( Aa ) that carried the potential to look like the recessive P 1 parent ( aa ). After observing this potential to express a trait without showing the phenotype, Mendel put forth his second principle of inheritance: the principle of segregation . According to this principle, the "particles" (or alleles as we now know them) that determine traits are separated into gametes during meiosis , and meiosis produces equal numbers of egg or sperm cells that contain each allele (Figure 5).
Dihybrid Crosses
Mendel had thus determined what happens when two plants that are hybrid for one trait are crossed with each other, but he also wanted to determine what happens when two plants that are each hybrid for two traits are crossed. Mendel therefore decided to examine the inheritance of two characteristics at once. Based on the concept of segregation , he predicted that traits must sort into gametes separately. By extrapolating from his earlier data, Mendel also predicted that the inheritance of one characteristic did not affect the inheritance of a different characteristic.
Mendel tested this idea of trait independence with more complex crosses. First, he generated plants that were purebred for two characteristics, such as seed color (yellow and green) and seed shape (round and wrinkled). These plants would serve as the P 1 generation for the experiment. In this case, Mendel crossed the plants with wrinkled and yellow seeds ( rrYY ) with plants with round, green seeds ( RRyy ). From his earlier monohybrid crosses, Mendel knew which traits were dominant: round and yellow. So, in the F 1 generation, he expected all round, yellow seeds from crossing these purebred varieties, and that is exactly what he observed. Mendel knew that each of the F 1 progeny were dihybrids; in other words, they contained both alleles for each characteristic ( RrYy ). He then crossed individual F 1 plants (with genotypes RrYy ) with one another. This is called a dihybrid cross . Mendel's results from this cross were as follows:
- 315 plants with round, yellow seeds
- 108 plants with round, green seeds
- 101 plants with wrinkled, yellow seeds
- 32 plants with wrinkled, green seeds
Thus, the various phenotypes were present in a 9:3:3:1 ratio (Figure 6).
Next, Mendel went through his data and examined each characteristic separately. He compared the total numbers of round versus wrinkled and yellow versus green peas, as shown in Tables 1 and 2.
Table 1: Data Regarding Seed Shape
Table 2: Data Regarding Pea Color
The proportion of each trait was still approximately 3:1 for both seed shape and seed color. In other words, the resulting seed shape and seed color looked as if they had come from two parallel monohybrid crosses; even though two characteristics were involved in one cross, these traits behaved as though they had segregated independently. From these data, Mendel developed the third principle of inheritance: the principle of independent assortment . According to this principle, alleles at one locus segregate into gametes independently of alleles at other loci. Such gametes are formed in equal frequencies.
Mendel’s Legacy
More lasting than the pea data Mendel presented in 1862 has been his methodical hypothesis testing and careful application of mathematical models to the study of biological inheritance. From his first experiments with monohybrid crosses, Mendel formed statistical predictions about trait inheritance that he could test with more complex experiments of dihybrid and even trihybrid crosses. This method of developing statistical expectations about inheritance data is one of the most significant contributions Mendel made to biology.
But do all organisms pass their on genes in the same way as the garden pea plant? The answer to that question is no, but many organisms do indeed show inheritance patterns similar to the seminal ones described by Mendel in the pea. In fact, the three principles of inheritance that Mendel laid out have had far greater impact than his original data from pea plant manipulations. To this day, scientists use Mendel's principles to explain the most basic phenomena of inheritance.
References and Recommended Reading
- Add Content to Group
Article History
Flag inappropriate.


Email your Friend

- | Lead Editor: Terry McGuire

Within this Subject (29)
- Gene Linkage (5)
- Methods for Studying Inheritance Patterns (7)
- The Foundation of Inheritance Studies (11)
- Variation in Gene Expression (6)
Other Topic Rooms
- Gene Inheritance and Transmission
- Gene Expression and Regulation
- Nucleic Acid Structure and Function
- Chromosomes and Cytogenetics
- Evolutionary Genetics
- Population and Quantitative Genetics
- Genes and Disease
- Genetics and Society
- Cell Origins and Metabolism
- Proteins and Gene Expression
- Subcellular Compartments
- Cell Communication
- Cell Cycle and Cell Division

© 2014 Nature Education
- Press Room |
- Terms of Use |
- Privacy Notice |

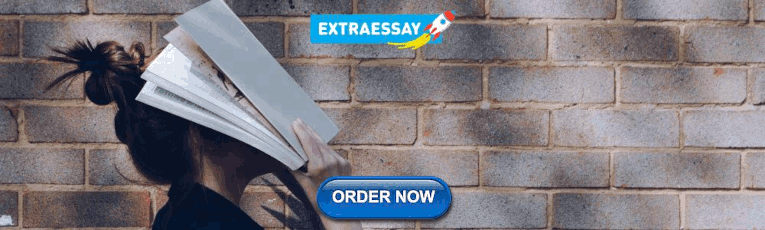
Visual Browse

Want to create or adapt books like this? Learn more about how Pressbooks supports open publishing practices.
21 Mendel’s Experiments
By the end of this section, you will be able to:
- Explain the scientific reasons for the success of Mendel’s experimental work
- Describe the expected outcomes of monohybrid crosses involving dominant and recessive alleles
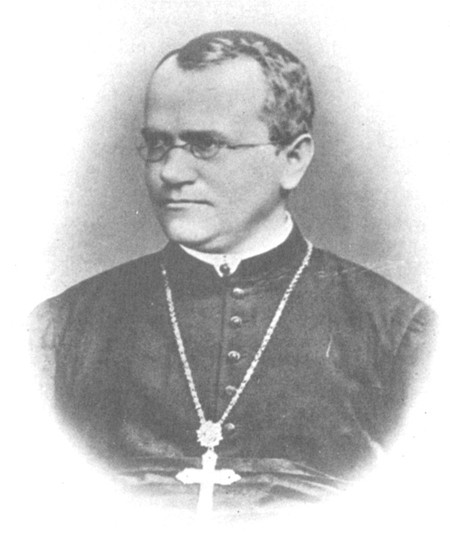
Johann Gregor Mendel (1822–1884) (Figure 1) was a lifelong learner, teacher, scientist, and man of faith. As a young adult, he joined the Augustinian Abbey of St. Thomas in Brno in what is now the Czech Republic. Supported by the monastery, he taught physics, botany, and natural science courses at the secondary and university levels. In 1856, he began a decade-long research pursuit involving inheritance patterns in honeybees and plants, ultimately settling on pea plants as his primary model system (a system with convenient characteristics that is used to study a specific biological phenomenon to gain understanding to be applied to other systems). In 1865, Mendel presented the results of his experiments with nearly 30,000 pea plants to the local natural history society. He demonstrated that traits are transmitted faithfully from parents to offspring in specific patterns. In 1866, he published his work, Experiments in Plant Hybridization, 1 in the proceedings of the Natural History Society of Brünn.
Mendel’s work went virtually unnoticed by the scientific community, which incorrectly believed that the process of inheritance involved a blending of parental traits that produced an intermediate physical appearance in offspring. This hypothetical process appeared to be correct because of what we know now as continuous variation. Continuous variation is the range of small differences we see among individuals in a characteristic like human height. It does appear that offspring are a “blend” of their parents’ traits when we look at characteristics that exhibit continuous variation. Mendel worked instead with traits that show discontinuous variation . Discontinuous variation is the variation seen among individuals when each individual shows one of two—or a very few—easily distinguishable traits, such as violet or white flowers. Mendel’s choice of these kinds of traits allowed him to see experimentally that the traits were not blended in the offspring as would have been expected at the time, but that they were inherited as distinct traits. In 1868, Mendel became abbot of the monastery and exchanged his scientific pursuits for his pastoral duties. He was not recognized for his extraordinary scientific contributions during his lifetime; in fact, it was not until 1900 that his work was rediscovered, reproduced, and revitalized by scientists on the brink of discovering the chromosomal basis of heredity.
Mendel’s Crosses
Mendel’s seminal work was accomplished using the garden pea, Pisum sativum , to study inheritance. This species naturally self-fertilizes, meaning that pollen encounters ova within the same flower. The flower petals remain sealed tightly until pollination is completed to prevent the pollination of other plants. The result is highly inbred, or “true-breeding,” pea plants. These are plants that always produce offspring that look like the parent. By experimenting with true-breeding pea plants, Mendel avoided the appearance of unexpected traits in offspring that might occur if the plants were not true-breeding. The garden pea also grows to maturity within one season, meaning that several generations could be evaluated over a relatively short time. Finally, large quantities of garden peas could be cultivated simultaneously, allowing Mendel to conclude that his results did not come about simply by chance.
Mendel performed hybridizations , which involve mating two true-breeding individuals that have different traits. In the pea, which is naturally self-pollinating, this is done by manually transferring pollen from the anther of a mature pea plant of one variety to the stigma of a separate mature pea plant of the second variety.
Plants used in first-generation crosses were called P , or parental generation, plants (Figure 2). Mendel collected the seeds produced by the P plants that resulted from each cross and grew them the following season. These offspring were called the F 1 , or the first filial (filial = daughter or son), generation. Once Mendel examined the characteristics in the F 1 generation of plants, he allowed them to self-fertilize naturally. He then collected and grew the seeds from the F 1 plants to produce the F 2 , or second filial, generation. Mendel’s experiments extended beyond the F 2 generation to the F 3 generation, F 4 generation, and so on, but it was the ratio of characteristics in the P, F 1 , and F 2 generations that were the most intriguing and became the basis of Mendel’s postulates.
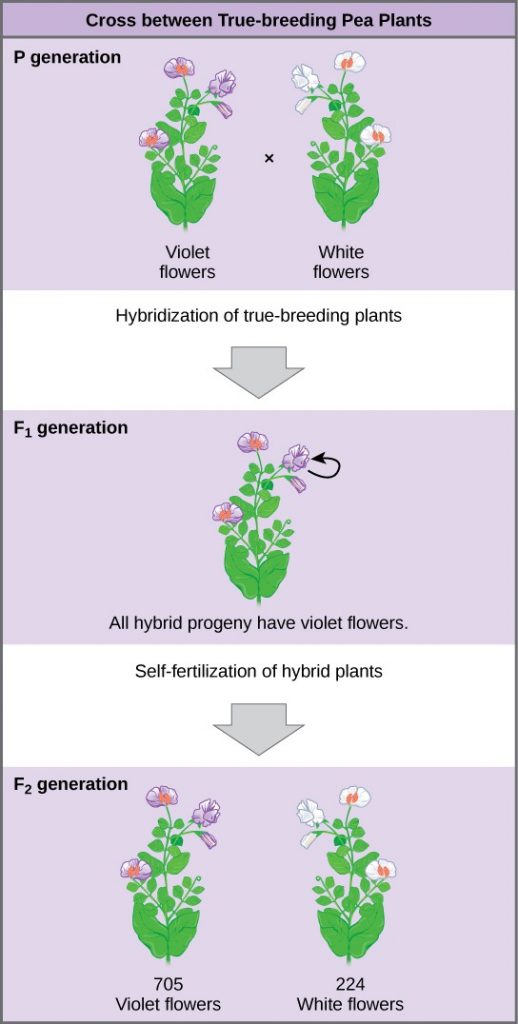
Garden Pea Characteristics Revealed the Basics of Heredity
In his 1865 publication, Mendel reported the results of his crosses involving seven different characteristics, each with two contrasting traits. A trait is defined as a variation in the physical appearance of a heritable characteristic. The characteristics included plant height, seed texture, seed color, flower color, pea-pod size, pea-pod color, and flower position. For the characteristic of flower color, for example, the two contrasting traits were white versus violet. To fully examine each characteristic, Mendel generated large numbers of F 1 and F 2 plants and reported results from thousands of F 2 plants.
What results did Mendel find in his crosses for flower color? First, Mendel confirmed that he was using plants that bred true for white or violet flower color. Irrespective of the number of generations that Mendel examined, all self-crossed offspring of parents with white flowers had white flowers, and all self-crossed offspring of parents with violet flowers had violet flowers. In addition, Mendel confirmed that, other than flower color, the pea plants were physically identical. This was an important check to make sure that the two varieties of pea plants only differed with respect to one trait, flower color.
Once these validations were complete, Mendel applied the pollen from a plant with violet flowers to the stigma of a plant with white flowers. After gathering and sowing the seeds that resulted from this cross, Mendel found that 100 percent of the F 1 hybrid generation had violet flowers. Conventional wisdom at that time would have predicted the hybrid flowers to be pale violet or for hybrid plants to have equal numbers of white and violet flowers. In other words, the contrasting parental traits were expected to blend in the offspring. Instead, Mendel’s results demonstrated that the white flower trait had completely disappeared in the F 1 generation.
Importantly, Mendel did not stop his experimentation there. He allowed the F 1 plants to self-fertilize and found that 705 plants in the F 2 generation had violet flowers and 224 had white flowers. This was a ratio of 3.15 violet flowers to one white flower, or approximately 3:1. When Mendel transferred pollen from a plant with violet flowers to the stigma of a plant with white flowers and vice versa, he obtained approximately the same ratio irrespective of which parent—male or female—contributed which trait. This is called a reciprocal cross —a paired cross in which the respective traits of the male and female in one cross become the respective traits of the female and male in the other cross. For the other six characteristics that Mendel examined, the F 1 and F 2 generations behaved in the same way that they behaved for flower color. One of the two traits would disappear completely from the F 1 generation, only to reappear in the F 2 generation at a ratio of roughly 3:1 (Figure 3).
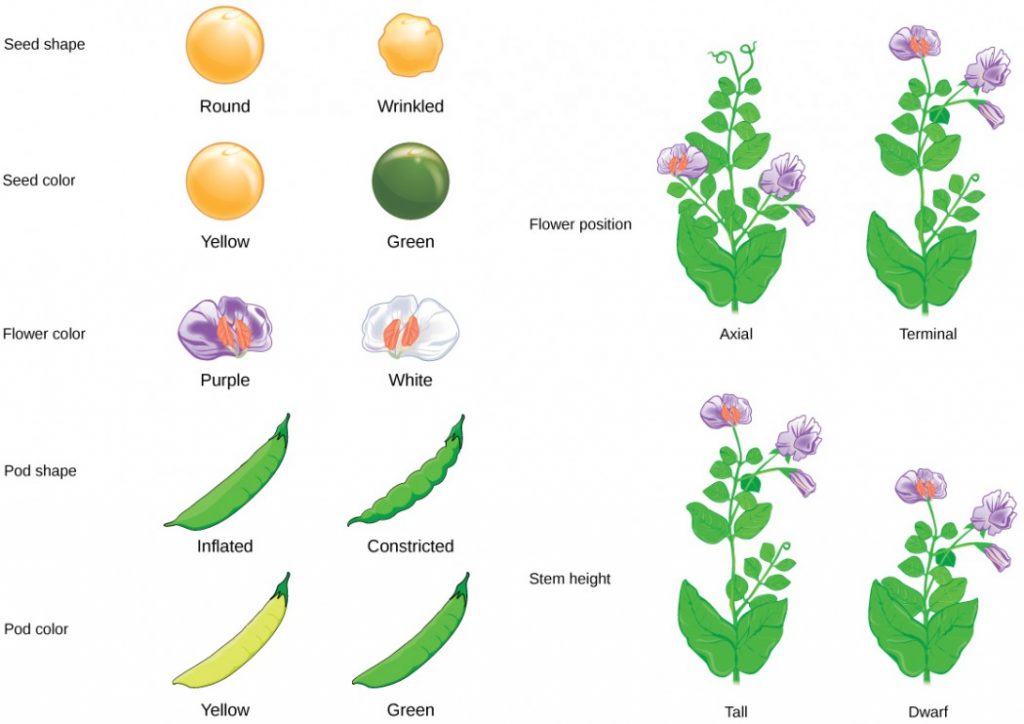
Upon compiling his results for many thousands of plants, Mendel concluded that the characteristics could be divided into expressed and latent traits. He called these dominant and recessive traits, respectively. Dominant traits are those that are inherited unchanged in a hybridization. Recessive traits become latent, or disappear in the offspring of a hybridization. The recessive trait does, however, reappear in the progeny of the hybrid offspring. An example of a dominant trait is the violet-colored flower trait. For this same characteristic (flower color), white-colored flowers are a recessive trait. The fact that the recessive trait reappeared in the F 2 generation meant that the traits remained separate (and were not blended) in the plants of the F 1 generation. Mendel proposed that this was because the plants possessed two copies of the trait for the flower-color characteristic, and that each parent transmitted one of their two copies to their offspring, where they came together. Moreover, the physical observation of a dominant trait could mean that the genetic composition of the organism included two dominant versions of the characteristic, or that it included one dominant and one recessive version. Conversely, the observation of a recessive trait meant that the organism lacked any dominant versions of this characteristic.
CONCEPTS IN ACTION
For an excellent review of Mendel’s experiments and to perform your own crosses and identify patterns of inheritance, visit the Mendel’s Peas web lab .
Also, check out the following video as review
- Johann Gregor Mendel, “Versuche über Pflanzenhybriden.” Verhandlungen des naturforschenden Vereines in Brünn , Bd. IV für das Jahr, 1865 Abhandlungen (1866):3–47. [for English translation, see http://www.mendelweb.org/Mendel.plain.html]
Introductory Biology: Evolutionary and Ecological Perspectives Copyright © by Various Authors - See Each Chapter Attribution is licensed under a Creative Commons Attribution 4.0 International License , except where otherwise noted.
Share This Book
Mendel's Experiments: The Study Of Pea Plants & Inheritance
** Gregor Mendel ** was a 19th-century pioneer of genetics who today is remembered almost entirely for two things: being a monk and relentlessly studying different traits of pea plants. Born in 1822 in Austria, Mendel was raised on a farm and attended the University of Vienna in Austria's capital city.
There, he studied science and math, a pairing that would prove invaluable to his future endeavors, which he conducted over an eight-year period entirely at the monastery where he lived.
In addition to formally studying the natural sciences in college, Mendel worked as a gardener in his youth and published research papers on the subject of crop damage by insects before taking up his now-famous work with Pisum sativum, the common pea plant. He maintained the monastery greenhouses and was familiar with the artificial fertilization techniques required to create limitless numbers of hybrid offspring.
An interesting historical footnote: While Mendel's experiments and those of the visionary biologist ** Charles Darwin ** both overlapped to a great extent, the latter never learned of Mendel's experiments.
Darwin formulated his ideas about inheritance without knowledge of Mendel's thoroughly detailed propositions about the mechanisms involved. Those propositions continue to inform the field of biological inheritance in the 21st century.
Understanding of Inheritance in the Mid-1800s
From the standpoint of basic qualifications, Mendel was perfectly positioned to make a major breakthrough in the then-all-but-nonexistent field of genetics, and he was blessed with both the environment and the patience to get done what he needed to do. Mendel would end up growing and studying nearly 29,000 pea plants between 1856 and 1863.
When Mendel first began his work with pea plants, the scientific concept of heredity was rooted in the concept of blended inheritance, which held that parental traits were somehow mixed into offspring in the manner of different-colored paints, producing a result that was not quite the mother and not quite the father every time, but that clearly resembled both.
Mendel was intuitively aware from his informal observation of plants that if there was any merit to this idea, it certainly didn't apply to the botanical world.
Mendel was not interested in the appearance of his pea plants per se. He examined them in order to understand which characteristics could be passed on to future generations and exactly how this occurred at a functional level, even if he didn't have the literal tools to see what was occurring at the molecular level.
Pea Plant Characteristics Studied
Mendel focused on the different traits, or characters, that he noticed pea plants exhibiting in a binary manner. That is, an individual plant could show either version A of a given trait or version B of that trait, but nothing in between. For example, some plants had "inflated" pea pods, whereas others looked "pinched," with no ambiguity as to which category a given plant's pods belonged in.
The seven traits Mendel identified as being useful to his aims and their different manifestations were:
• Flower color: Purple or white. • Flower position: Axial (along the side of the stem) or terminal (at the end of the stem). • Stem length: Long or short. • Pod shape: Inflated or pinched. • Pod color: Green or yellow. • Seed shape: Round or wrinkled. • Seed color: Green or yellow.
Pea Plant Pollination
Pea plants can self-pollinate with no help from people. As useful as this is to plants, it introduced a complication into Mendel's work. He needed to prevent this from happening and allow only cross-pollination (pollination between different plants), since self-pollination in a plant that does not vary for a given trait does not provide helpful information.
In other words, he needed to control what characteristics could show up in the plants he bred, even if he didn't know in advance precisely which ones would manifest themselves and in what proportions.
Mendel's First Experiment
When Mendel began to formulate specific ideas about what he hoped to test and identify, he asked himself a number of basic questions. For example, what would happen when plants that were true-breeding for different versions of the same trait were cross-pollinated?
"True-breeding" means capable of producing one and only one type of offspring, such as when all daughter plants are round-seeded or axial-flowered. A true line shows no variation for the trait in question throughout a theoretically infinite number of generations, and also when any two selected plants in the scheme are bred with each other.
• To be certain his plant lines were true, Mendel spent two years creating them.
If the idea of blended inheritance were valid, blending a line of, say, tall-stemmed plants with a line of short-stemmed plants should result in some tall plants, some short plants and plants along the height spectrum in between, rather like humans. Mendel learned, however, that this did not happen at all. This was both confounding and exciting.
Mendel's Generational Assessment: P, F1, F2
Once Mendel had two sets of plants that differed only at a single trait, he performed a multigenerational assessment in an effort to try to follow the transmission of traits through multiple generations. First, some terminology:
• The parent generation was the P generation , and it included a P1 plant whose members all displayed one version of a trait and a P2 plant whose members all displayed the other version. • The hybrid offspring of the P generation was the F1 (filial) generation . • The offspring of the F1 generation was the F2 generation (the "grandchildren" of the P generation).
This is called a _ monohybrid cross _: "mono" because only one trait varied, and "hybrid" because offspring represented a mixture, or hybridization, of plants, as one parent has one version of the trait while one had the other version.
For the present example, this trait will be seed shape (round vs. wrinkled). One could also use flower color (white vs. purpl) or seed color (green or yellow).
Mendel's Results (First Experiment)
Mendel assessed genetic crosses from the three generations to assess the heritability of characteristics across generations. When he looked at each generation, he discovered that for all seven of his chosen traits, a predictable pattern emerged.
For example, when he bred true-breeding round-seeded plants (P1) with true-breeding wrinkled-seeded plants (P2):
• All of the plants in the F1 generation had round seeds . This seemed to suggest that the wrinkled trait had been obliterated by the round trait. • However, he also found that, while about three-fourths of the plants in the F2 generation has round seeds, about one-fourth of these plants had wrinkled seeds . Clearly, the wrinkled trait had somehow "hidden" in the F1 generation and re-emerged in the F2 generation.
This led to the concept of dominant traits (here, round seeds) and recessive traits (in this case, wrinkled seeds).
This implied that the plants' _ phenotype (what the plants actually looked like) was not a strict reflection of their genotype _ (the information that was actually somehow coded into the plants and passed along to subsequent generations).
Mendel then produced some formal ideas to explain this phenomenon, both the mechanism of heritability and the mathematical ratio of a dominant trait to a recessive trait in any circumstance where the composition of allele pairs is known.
Mendel's Theory of Heredity
Mendel crafted a theory of heredity that consisted of four hypotheses:
1. Genes (a gene being the chemical code for a given trait) can come in different types. 2. For each characteristic, an organism inherits one allele (version of a gene) from each parent. 3. When two different alleles are inherited, one may be expressed while the other is not. 4. When gametes (sex cells, which in humans are sperm cells and egg cells) are formed, the two alleles of each gene are separated.
The last of these represents the ** law of segregation **, stipulating that the alleles for each trait separate randomly into the gametes.
Today, scientists recognize that the P plants that Mendel had "bred true" were homozygous for the trait he was studying: They had two copies of the same allele at the gene in question.
Since round was clearly dominant over wrinkled, this can be represented by RR and rr, as capital letters signify dominance and lowercase letters indicate recessive traits. When both alleles are present, the trait of the dominant allele was manifested in its phenotype.
The Monohybrid Cross Results Explained
Based on the foregoing, a plant with a genotype RR at the seed-shape gene can only have round seeds, and the same is true of the Rr genotype, as the "r" allele is masked. Only plants with an rr genotype can have wrinkled seeds.
And sure enough, the four possible combinations of genotypes (RR, rR, Rr and rr) yield a 3:1 phenotypic ratio, with about three plants with round seeds for every one plant with wrinkled seeds.
Because all of the P plants were homozygous, RR for the round-seed plants and rr for the wrinkled-seed plants, all of the F1 plants could only have the genotype Rr. This meant that while all of them had round seeds, they were all carriers of the recessive allele, which could therefore appear in subsequent generations thanks to the law of segregation.
This is precisely what happened. Given F1 plants that all had an Rr genotype, their offspring (the F2 plants) could have any of the four genotypes listed above. The ratios were not exactly 3:1 owing to the randomness of the gamete pairings in fertilization, but the more offspring that were produced, the closer the ratio came to being exactly 3:1.
Mendel's Second Experiment
Next, Mendel created _ dihybrid crosses _, wherein he looked at two traits at once rather than just one. The parents were still true-breeding for both traits, for example, round seeds with green pods and wrinkled seeds with yellow pods, with green dominant over yellow. The corresponding genotypes were therefore RRGG and rrgg.
As before, the F1 plants all looked like the parent with both dominant traits. The ratios of the four possible phenotypes in the F2 generation (round-green, round-yellow, wrinkled-green, wrinkled-yellow) turned out to be 9:3:3:1
This bore out Mendel's suspicion that different traits were inherited independently of one another, leading him to posit the ** law of independent assortment **. This principle explains why you might have the same eye color as one of your siblings, but a different hair color; each trait is fed into the system in a manner that is blind to all of the others.
Linked Genes on Chromosomes
Today, we know the real picture is a little more complicated, because in fact, genes that happen to be physically close to each other on chromosomes can be inherited together thanks to chromosome exchange during gamete formation.
In the real world, if you looked at limited geographical areas of the U.S., you would expect to find more New York Yankees and Boston Red Sox fans in close proximity than either Yankees-Los Angeles Dodgers fans or Red Sox-Dodgers fans in the same area, because Boston and New York are close together and both are close to 3,000 miles from Los Angeles.
Mendelian Inheritance
As it happens, not all traits obey this pattern of inheritance. But those that do are called Mendelian traits . Returning to the dihybrid cross mentioned above, there are sixteen possible genotypes:
RRGG, RRgG, RRGg, RRgg, RrGG, RrgG, RrGg, Rrgg, rRGG, rRgG, rRGg, rRgg, rrGG, rrGg, rrgG, rrgg
When you work out the phenotypes, you see that the probability ratio of
round green, round yellow, wrinkled green, wrinkled yellow
turns out to be 9:3:3:1. Mendel's painstaking counting of his different plant types revealed that the ratios were close enough to this prediction for him to conclude that his hypotheses were correct.
• Note: A genotype of rR is functionally equivalent to Rr. The only difference is which parent contributes which allele to the mix.
- Scitable by Nature Education: Gregor Mendel and the Principles of Inheritance
- Biology LibreTexts: Mendel's Pea Plants
- OpenText BC: Concepts of Biology: Laws of Inheritance
- Forbes Magazine: How Mendel Channeled Darwin
Cite This Article
Beck, Kevin. "Mendel's Experiments: The Study Of Pea Plants & Inheritance" sciencing.com , https://www.sciencing.com/mendels-experiments-the-study-of-pea-plants-inheritance-13718433/. 8 May 2019.
Beck, Kevin. (2019, May 8). Mendel's Experiments: The Study Of Pea Plants & Inheritance. sciencing.com . Retrieved from https://www.sciencing.com/mendels-experiments-the-study-of-pea-plants-inheritance-13718433/
Beck, Kevin. Mendel's Experiments: The Study Of Pea Plants & Inheritance last modified August 30, 2022. https://www.sciencing.com/mendels-experiments-the-study-of-pea-plants-inheritance-13718433/
Recommended
Gregor Mendel
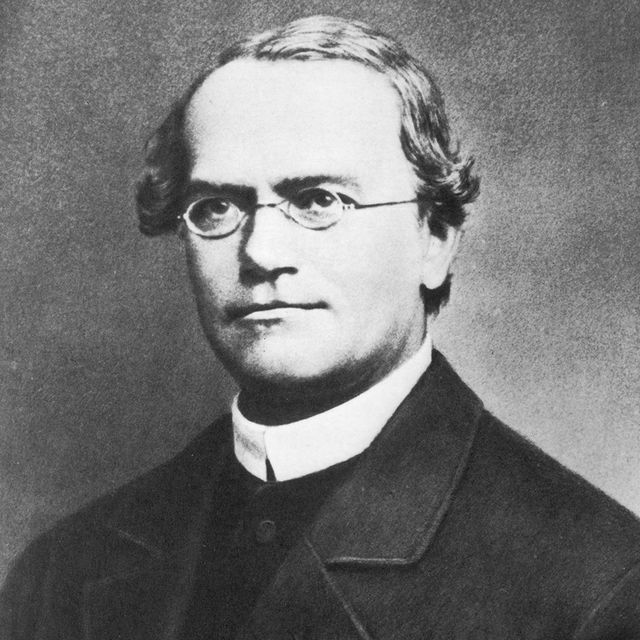
(1822-1884)
Who Was Gregor Mendel?
Gregor Mendel, known as the "father of modern genetics," was born in Austria in 1822. A monk, Mendel discovered the basic principles of heredity through experiments in his monastery's garden. His experiments showed that the inheritance of certain traits in pea plants follows particular patterns, subsequently becoming the foundation of modern genetics and leading to the study of heredity.
Gregor Johann Mendel was born Johann Mendel on July 20, 1822, to Anton and Rosine Mendel, on his family’s farm, in what was then Heinzendorf, Austria. He spent his early youth in that rural setting, until age 11, when a local schoolmaster who was impressed with his aptitude for learning recommended that he be sent to secondary school in Troppau to continue his education. The move was a financial strain on his family, and often a difficult experience for Mendel, but he excelled in his studies, and in 1840, he graduated from the school with honors.
Following his graduation, Mendel enrolled in a two-year program at the Philosophical Institute of the University of Olmütz. There, he again distinguished himself academically, particularly in the subjects of physics and math, and tutored in his spare time to make ends meet. Despite suffering from deep bouts of depression that, more than once, caused him to temporarily abandon his studies, Mendel graduated from the program in 1843.
That same year, against the wishes of his father, who expected him to take over the family farm, Mendel began studying to be a monk: He joined the Augustinian order at the St. Thomas Monastery in Brno, and was given the name Gregor. At that time, the monastery was a cultural center for the region, and Mendel was immediately exposed to the research and teaching of its members, and also gained access to the monastery’s extensive library and experimental facilities.
In 1849, when his work in the community in Brno exhausted him to the point of illness, Mendel was sent to fill a temporary teaching position in Znaim. However, he failed a teaching-certification exam the following year, and in 1851, he was sent to the University of Vienna, at the monastery’s expense, to continue his studies in the sciences. While there, Mendel studied mathematics and physics under Christian Doppler, after whom the Doppler effect of wave frequency is named; he studied botany under Franz Unger, who had begun using a microscope in his studies, and who was a proponent of a pre-Darwinian version of evolutionary theory.
In 1853, upon completing his studies at the University of Vienna, Mendel returned to the monastery in Brno and was given a teaching position at a secondary school, where he would stay for more than a decade. It was during this time that he began the experiments for which he is best known.
Experiments and Theories
Around 1854, Mendel began to research the transmission of hereditary traits in plant hybrids. At the time of Mendel’s studies, it was a generally accepted fact that the hereditary traits of the offspring of any species were merely the diluted blending of whatever traits were present in the “parents.” It was also commonly accepted that, over generations, a hybrid would revert to its original form, the implication of which suggested that a hybrid could not create new forms. However, the results of such studies were often skewed by the relatively short period of time during which the experiments were conducted, whereas Mendel’s research continued over as many as eight years (between 1856 and 1863), and involved tens of thousands of individual plants.
Mendel chose to use peas for his experiments due to their many distinct varieties, and because offspring could be quickly and easily produced. He cross-fertilized pea plants that had clearly opposite characteristics—tall with short, smooth with wrinkled, those containing green seeds with those containing yellow seeds, etc.—and, after analyzing his results, reached two of his most important conclusions: the Law of Segregation, which established that there are dominant and recessive traits passed on randomly from parents to offspring (and provided an alternative to blending inheritance, the dominant theory of the time), and the Law of Independent Assortment, which established that traits were passed on independently of other traits from parent to offspring. He also proposed that this heredity followed basic statistical laws. Though Mendel’s experiments had been conducted with pea plants, he put forth the theory that all living things had such traits.
In 1865, Mendel delivered two lectures on his findings to the Natural Science Society in Brno, who published the results of his studies in their journal the following year, under the title Experiments on Plant Hybrids . Mendel did little to promote his work, however, and the few references to his work from that time period indicated that much of it had been misunderstood. It was generally thought that Mendel had shown only what was already commonly known at the time—that hybrids eventually revert to their original form. The importance of variability and its evolutionary implications were largely overlooked. Furthermore, Mendel's findings were not viewed as being generally applicable, even by Mendel himself, who surmised that they only applied to certain species or types of traits. Of course, his system eventually proved to be of general application and is one of the foundational principles of biology.
Later Life, Death and Legacy
In 1868, Mendel was elected abbot of the school where he had been teaching for the previous 14 years, and both his resulting administrative duties and his gradually failing eyesight kept him from continuing any extensive scientific work. He traveled little during this time and was further isolated from his contemporaries as the result of his public opposition to an 1874 taxation law that increased the tax on the monasteries to cover Church expenses.
Gregor Mendel died on January 6, 1884, at the age of 61. He was laid to rest in the monastery’s burial plot and his funeral was well attended. His work, however, was still largely unknown.
It was not until decades later, when Mendel’s research informed the work of several noted geneticists, botanists and biologists conducting research on heredity, that its significance was more fully appreciated, and his studies began to be referred to as Mendel’s Laws. Hugo de Vries, Carl Correns and Erich von Tschermak-Seysenegg each independently duplicated Mendel's experiments and results in 1900, finding out after the fact, allegedly, that both the data and the general theory had been published in 1866 by Mendel. Questions arose about the validity of the claims that the trio of botanists were not aware of Mendel's previous results, but they soon did credit Mendel with priority. Even then, however, his work was often marginalized by Darwinians, who claimed that his findings were irrelevant to a theory of evolution. As genetic theory continued to develop, the relevance of Mendel’s work fell in and out of favor, but his research and theories are considered fundamental to any understanding of the field, and he is thus considered the "father of modern genetics."
QUICK FACTS
- Name: Gregor Mendel
- Birth Year: 1822
- Birth date: July 20, 1822
- Birth City: Heinzendorf
- Birth Country: Austria
- Gender: Male
- Best Known For: Gregor Mendel was an Austrian monk who discovered the basic principles of heredity through experiments in his garden. Mendel's observations became the foundation of modern genetics and the study of heredity, and he is widely considered a pioneer in the field of genetics.
- Science and Medicine
- Astrological Sign: Cancer
- University of Vienna
- University of Olmütz
- Nacionalities
- Death Year: 1884
- Death date: January 6, 1884
- Death City: Brno
- Death Country: Austria
We strive for accuracy and fairness.If you see something that doesn't look right, contact us !
CITATION INFORMATION
- Article Title: Gregor Mendel Biography
- Author: Biography.com Editors
- Website Name: The Biography.com website
- Url: https://www.biography.com/scientists/gregor-mendel
- Access Date:
- Publisher: A&E; Television Networks
- Last Updated: May 21, 2021
- Original Published Date: April 2, 2014
- My scientific studies have afforded me great gratification; and I am convinced that it will not be long before the whole world acknowledges the results of my work.
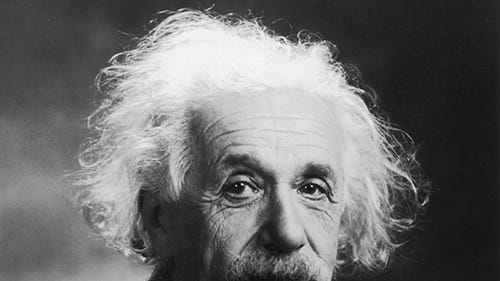
Famous Scientists
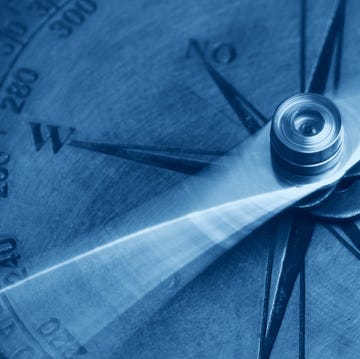
Did This Newly Found Compass Belong to Copernicus?
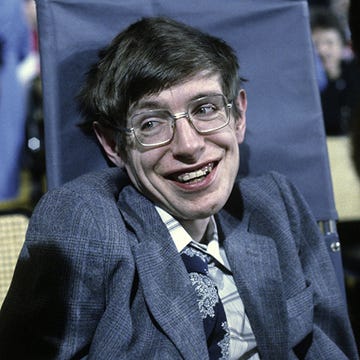
Stephen Hawking
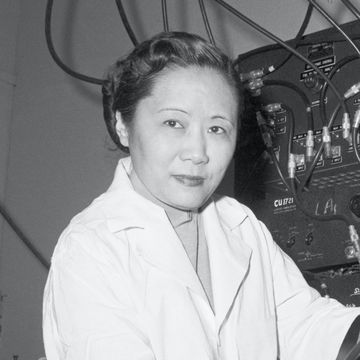
Chien-Shiung Wu
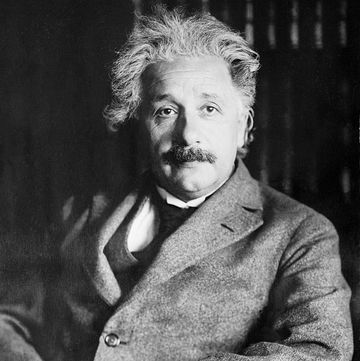
The Solar Eclipse That Made Albert Einstein a Star
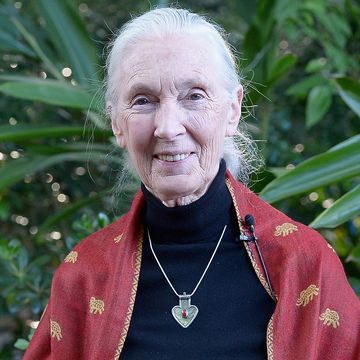
Jane Goodall
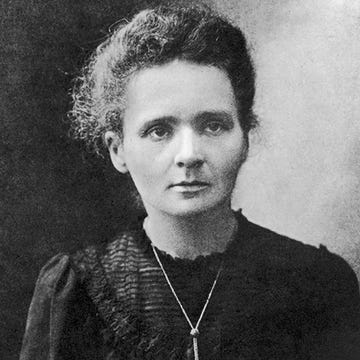
Marie Curie
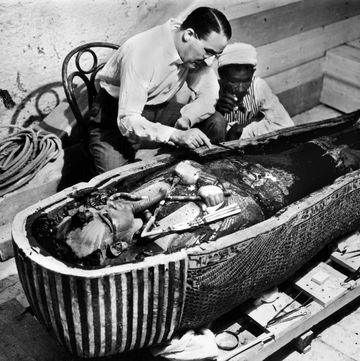
Howard Carter, King Tut's Tomb, and a Deadly Curse
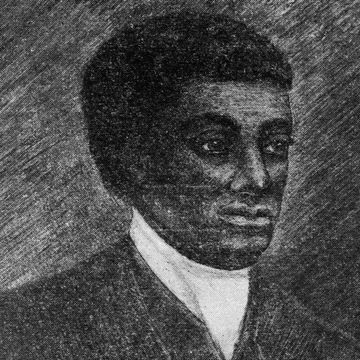
Benjamin Banneker
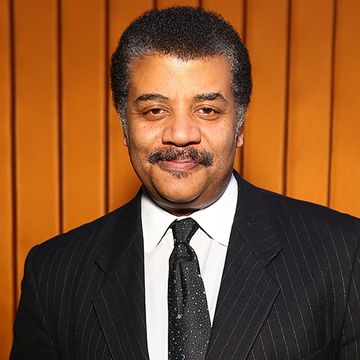
Neil deGrasse Tyson
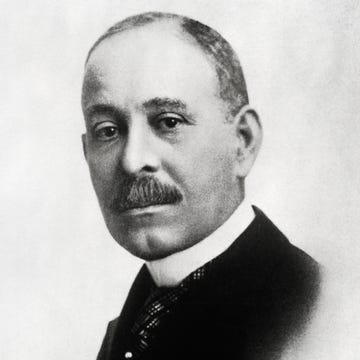
Daniel Hale Williams
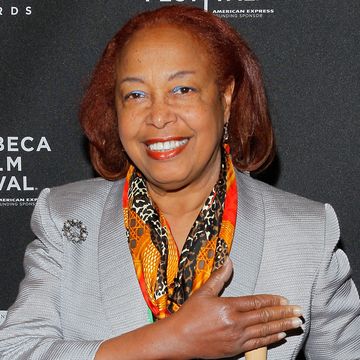
Patricia Bath
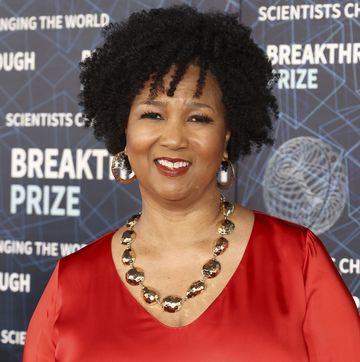
Mae Jemison
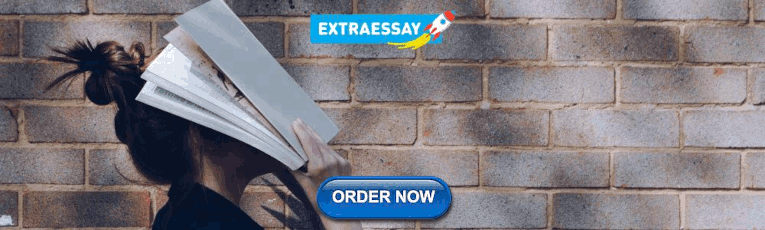
IMAGES
VIDEO
COMMENTS
In 1856, Mendel began a series of experiments at the monastery to find out how traits are passed from generation to generation. At the time, it was thought that parents' traits were blended together in their progeny. Studying traits in peas. Mendel studied inheritance in peas (Pisum sativum). He chose peas because they had been used for ...
Learn about Mendel's experiments on pea plants and his laws of inheritance: dominance, segregation and independent assortment. Find out how genes are transferred from parents to offspring and how traits are expressed and inherited.
Learn how Mendel used pea plant breeding to discover the laws of inheritance of dominant and recessive traits. Explore the methods, observations, and conclusions of his famous experiments and their impact on modern genetics.
Mendel's experiments extended beyond the F 2 generation to the F 3 generation, F 4 generation, and so on, but it was the ratio of characteristics in the P, F 1, and F 2 generations that were the most intriguing and became the basis of Mendel's postulates. Figure 2: Mendel's process for performing crosses included examining flower color.
Mendelian inheritance is a term arising from the singular work of the 19th-century scientist and Austrian monk Gregor Mendel. His experiments on pea plants highlighted the mechanisms of inheritance in organisms that reproduce sexually and led to the laws of segregation and independent assortment.
Learn how Gregor Mendel used pea plants to discover the secrets of heredity, or how parents pass characteristics to their offspring. Explore his experiments, observations, and laws of segregation and independent assortment.
Learn about Gregor Mendel's experiments with pea plants and how they led to the principles of inheritance.
Gregor Mendel (born July 20, 1822, Heinzendorf, Silesia, Austrian Empire [now Hynčice, Czech Republic]—died January 6, 1884, Brünn, Austria-Hungary [now Brno, Czech Republic]) was a botanist, teacher, and Augustinian prelate, the first person to lay the mathematical foundation of the science of genetics, in what came to be called Mendelism.
Gregor Mendel was an Austrian monk who discovered the basic principles of heredity through experiments in his garden. Mendel's observations became the foundation of modern genetics and the study ...
by Mendel, including smooth or wrinkled ripe seeds, yellow or green seed albumen, purple or white flower, tall or dwarf stem length, and others. WHAT WERE THE FINDINGS? Over the course of his experiments, Mendel made three important discoveries: 1. The Law of Segregation: offspring acquire one hereditary factor from each parent 2.